Renewables in remote mines – a litmus test for the wider renewables transition
Entura’s Greg Koppens has recently returned from the Energy and Mines Summit in Perth, where he led the ‘Think Tank’: a collaborative session addressing the challenge of powering process steam requirements from renewables-generated electricity. Here he shares his observations on the rise of renewables in the mining industry …
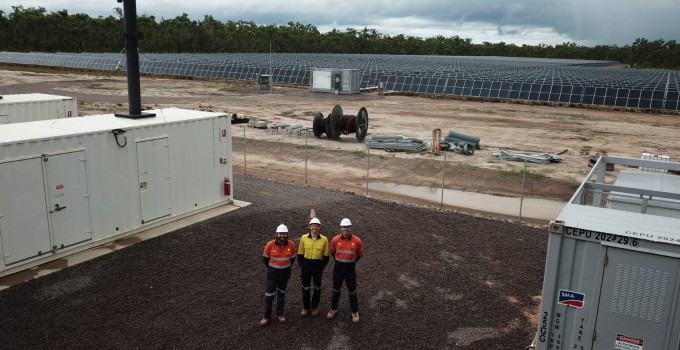
Australia’s mining industry is beginning the peak phase of its energy revolution. However, I find it unfortunate that this exciting fact is invisible to regular people. It is happening in remote areas on mining leases, inaccessible to the public. Each project alone is not sensational enough for media attention, but added together these projects are nothing short of a technology revolution. For remote mining sites to have a solar farm is now standard practice. In many cases this is backed up by battery storage and a handful of mines have onsite wind turbines.
The past: fossil-fuel driven mines
Australia is world-renowned for our mining industries, with mines of virtually every resource throughout our country. Up until about ten years ago, nearly all of Australia’s mines ran completely on diesel or gas. In most cases diesel was trucked in, or gas was brought in via pipeline. Most of these mines have energy expenses in the tens of millions of dollars per year. A small change in the oil price can drastically impact the mine’s bottom line.
If there’s one doubtless fact about mining, it’s that miners are practical people. They are problem solvers, and they know what works. With the boom-and-bust nature of resource markets and shareholder responsibilities, finances must be well managed.
The present: hybrid renewables power generation
At the Energy and Mines Summit, there was no discussion about whether it’s a good idea to consider renewables in the mix of power generation – it was simply a given. This is an industry in ‘early maturity’: it is no longer pioneers running a trial. Several systems are in the order of 100 MW capacity. Renewables are a tested and proven business decision. Everyone has crunched the numbers on their sites and, while each project has unique site-specific requirements, the conclusions are unanimous. The frontier is now getting access to skilled people, there is community engagement for siting of large assets and mutual benefits, and the industry is exploring emerging technologies such as electric fleet.
We now have all the technology needed to harness the power of the wind and the sun in an 80/20 mix with fossil fuels. Or a 50/50 mix when the system has solar power alone. And this is what is being implemented in practice all over Australia. We can do it in a way that achieves targets for price, reliability, service life, operability, maintainability and environmental impact. This greatly reduces the costs and risks of intermediate services such as refining and transportation as well as exposure to the global oil price. Mining companies are taking control of their energy supply by either owning the energy and storage assets or building well-defined low-risk partnerships.
The big project: transitioning the Pilbara to renewables
At the conference there was some focus on the Pilbara, which is a huge and complicated energy consumer, consuming 16 TWh (16 million MWh) of electricity per year, mostly coming from gas.
On the surface, it seems to be very low hanging fruit to quickly construct a few solar and wind assets to shift this picture. However, in the interests of the best long-term outcomes, the area needs planning, consultation and coordination. The Western Australian Government has developed a plan for ensuring that common-use infrastructure is used where possible, rather than risking having multiple redundant assets owned by different corporations. Aboriginal and community participation is recognised as crucial for the appropriate siting of wind, solar and transmission equipment.
The near future: electric fleet
Approximately half of a typical mine’s energy needs can be met by onsite electricity generation. The other half currently requires diesel fuel to run a fleet of light vehicles, monstrous ‘haul’ dump trucks such as the 250 tonne CAT 793, and every piece of mobile machinery in between. These mobile machines are the next target for reducing costs, carbon emissions and labour. Several trial projects are happening. For underground mines, where diesel has previously been used, huge ventilation fans will need far less power when there are no exhaust fumes to expel.
Machine manufacturers are developing a wide array of specialised battery electric products. In some applications, there is battery swap technology, as in modern power tools. In other applications, a fixed battery plugs in to recharge, like in an electric car. There are also trolley systems, akin to a tram or train. The best system for each job depends on the application.
Transitioning to a fully electric fleet will significantly increase a site’s electricity needs, with the biggest chargers running at 6 MW at full power. We are expecting to see sites’ electrical grids upgraded soon to integrate this high-power charging.
What is Entura doing?
At Entura, in addition to electrical generation and distribution design, we have specialised capabilities in control systems and power system studies for mining projects. Our microgrid control system (MCS) uses standard and reliable industrial Allen Bradley hardware to monitor and control the power station assets. We have a field-proven core algorithm for maintaining a priority of reliable power supply including backup/reserve supply, while making the greatest use of wind and solar where available. We’ve proven this process in our existing installations in the field and we lead the industry in methodology, practicality and voltage/frequency management.
We’ve seen the real benefits these systems bring to our clients and communities, both at mining sites and in other remote locations. Entura has a long history of design, formation and operation of microgrids throughout Australia (such as King Island, Flinders Island, Rottnest Island, and at mining sites such as the Agnew gold mine) and in the Pacific region (including the Cook Islands, the Federated States of Micronesia, Tonga, and the Solomon Islands, to name just a few).
Contact us if you’re interested in unlocking the full potential of microgrids for your operation or community, or if you’re interested in ways to increase your use of renewable energy.
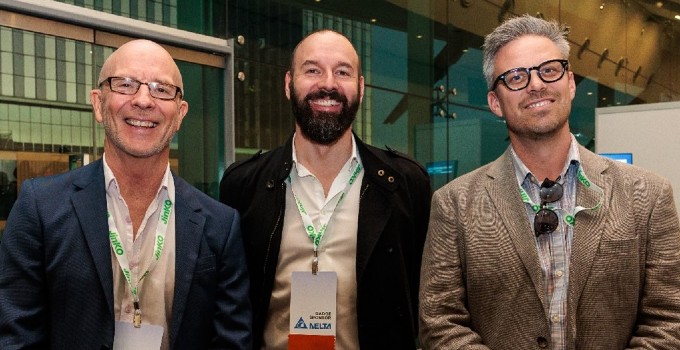
[Image immediately above] Entura’s Patrick Pease, Greg Koppens and Mark Richardson at the 2025 Energy and Mines Summit
[Top of article] Greg Koppens (centre) onsite at Jabiru Power Station, Northern Territory
ABOUT THE AUTHOR
Greg Koppens is Entura’s Principal Control Engineer Hybrid Renewable Systems and previously led Entura’s secondary electrical engineering team. Greg’s experience spans power, oil and gas, and mining, including onsite roles. With over two decades of detailed design experience, Greg facilitates collaboration between engineering disciplines and other stakeholders to solve complex problems. He regularly shares his extensive expertise with the mining sector to advance their decarbonisation goals. Find out more about Greg in our podcast series here.
New technologies are important tools, but they need to be used properly
Entura’s Technical Director (Water), Richard Herweynen, recently attended the 2025 ICOLD Congress in Chengdu, China, themed ‘Common Challenges, Shared Future, Better Dams’. Here he shares his observations on the state of play in the international dams industry – and the opportunities emerging with artificial intelligence and automation.
My first ICOLD Congress was in Beijing, China in 2000. I was presenting some finite element analysis work that I had done on Gordon Dam, a 140m-high concrete arch dam in Tasmania. The analysis was being used to help predict and explain some cracks that had formed at the base of the downstream face of the concrete shell, roughly normal to the foundation, during first filling. In the 1980s, an attempt had been made to model the crack using finite element modelling, but with little success due to the coarseness of the mesh. However, by the late 1990s, computing power had increased and finite element programs had improved, providing the capability to construct more detailed finite element models, which were able to predict and explain the cracking that had occurred.
Dr Sergio Giudici, the designer of Gordon Dam, was my mentor on this finite element analysis, and he reinforced these principles:
- It is important to verify the input data to make sure the model represents, as well as possible, the actual dam parameters.
- Results should be validated using alternative techniques to give the engineer confidence in the results that the model is producing (i.e. structural hand calculations still have a place).
- Complexity should be built into these models only gradually, so that the engineer can see the impact of changes and determine whether they are reasonable.
These same principles are true for many complex engineering models and when setting up calculation spreadsheets or similar.
Now, 25 years on, I have had the privilege of attending the 2025 ICOLD Congress in Chengdu, China. At this Congress, there was much talk about the importance of dams in society for water security, the growing role dams and reservoirs play in providing resilience to climate change and the energy transition, the importance of balancing economic benefits and environmental needs, and – in all of this – the importance of ensuring that our dams are safe for communities downstream.
We have discussed many of these themes before – and they remain highly important; however, one thing I took away from ICOLD 2025 in particular was how China is embracing technology and advancing a ‘Smart Dam’ initiative.
New technologies and smarter dams
One could say that our industry has always been building smart dams, but China’s ‘Smart Dam’ concept is about using the full power of current technologies to construct, monitor and operate dams in smarter ways, and to use technologies to predict and adapt to changing conditions.
The CHINCOLD Workshop on Digital and Intelligent Technologies for Dam Construction, Operation and Maintenance, which occurred during ICOLD 2025, gave a glimpse of what may be possible.
Many projects utilise 3-dimensional digital models and building information modelling (BIM). However, the concept of having a digital twin of the dam, replicating every aspect of the physical dam in a digital form, opens the door to many possibilities, especially in light of the advent of artificial intelligence (AI).
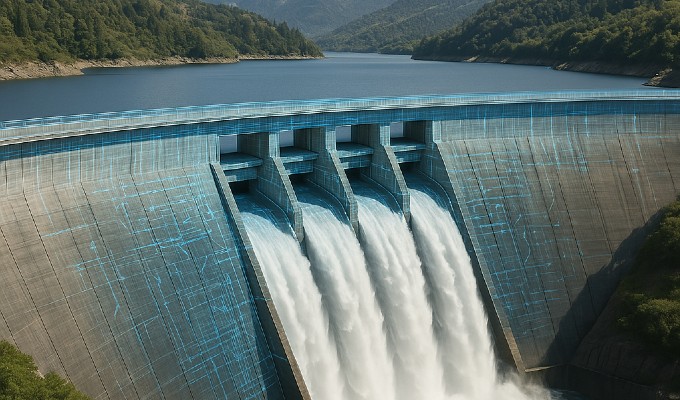
Artificial intelligence and machine learning
Many organisations and nations have been cautious about AI, but China is increasingly adopting it to improve construction practices in dam engineering, improve monitoring and surveillance of dams, and to help adapt to extreme events as they occur.
Machine learning (ML), an element of AI, enables computers to learn from data without being explicitly programmed. ML algorithms analyse data, identify patterns, and make predictions or decisions, improving their performance over time and with more data. There are no doubt many engineering applications where ML could be used to help improve predictive modelling or optimisation, and to make these more efficient. However, the same principles that were important when we were beginning to embrace larger, more refined finite element models with the advent of faster computing remain true here:
- We must verify the input data that machine learning is utilising to ensure we don’t get ‘garbage in equals garbage out’.
- Results need to be validated. AI needs to be trained correctly, and we need experts involved at this stage to ensure that the outputs from AI are correct.
- Complexity, or the full power of technology, should be added incrementally, to provide progressive confidence in the outcomes.
Engineering is the application of science, and therefore it is critical for every engineer to understand the fundamental principles and how to apply them. The complex computer programs used for a lot of engineering modelling can become ‘black boxes’, and practitioners risk diluting or losing their understanding of the fundamental principles behind the models, and hence their ability to validate the results.
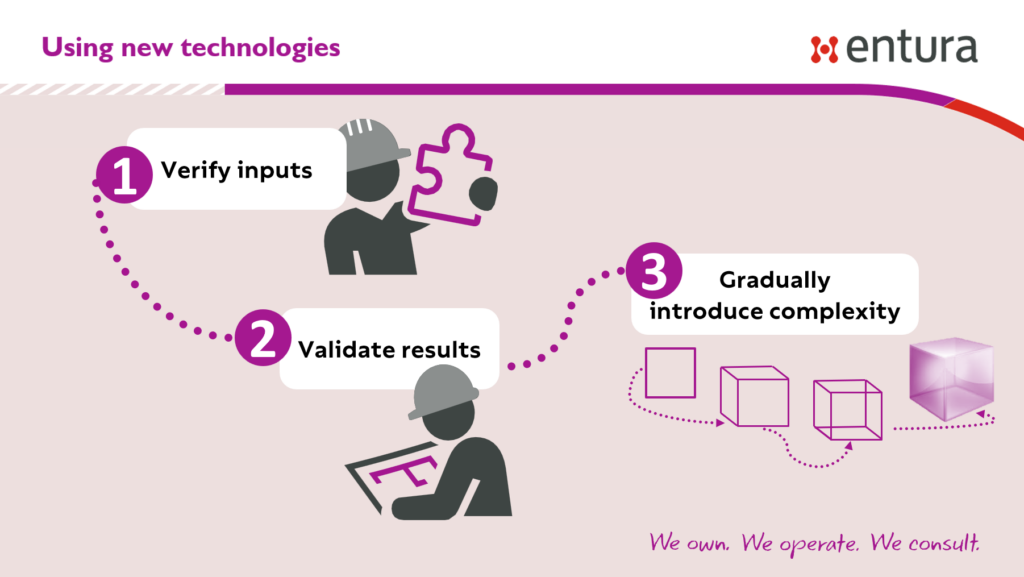
Automation brings a step change in efficiency and accuracy
At the ICOLD Congress in 2000, I co-authored a paper for the international symposium on concrete-faced rockfill dams (CFRD) entitled ‘Hydro Tasmania experience in concrete-faced rockfill dams – past, present and future’. There is no doubt that Hydro Tasmania has a strong history in CFRD, with Cethana Dam playing an important role in the development of the modern, high CFRD.
In 2000, however, the future in CFRD that we envisaged did not include unmanned construction equipment with automatic quality control feedback loops. At the 2025 CHINCOLD Workshop on Digital and Intelligent Technologies for Dam Construction, Operation and Maintenance, a presentation was given by Wang Jiajun from Tianjin University on intelligent unmanned roller systems to compact rockfill dams. Unmanned rolling compaction (URC) systems involve three core technology modules: (1) intelligent perception, (2) autonomous planning and decision making, and (3) intelligent control. These systems use automated driving technology to control the rolling process on earth and rockfill dams, improving productivity and quality. They can accurately control compaction parameters such as passes, speed, vibration and lift thickness. URC systems also enable continuous monitoring and real-time feedback for quality control, reducing human error and improving overall project performance. This automated technology was used on the 295m-high, 1.57km-long Lianghekou hydropower dam in China, with a total fill volume of 43 million m3.
The changing face of dam engineering
With AI and automation, the scope for embracing new technology in dam engineering is growing fast. It is clear that there are significant benefits that could be realised for dam design, construction, operation, dam safety and emergency response – and there’s a role for these advanced technologies at all of the stages of the life cycle of a dam. A degree of caution is appropriate and necessary, but caution should not be a reason to refuse to engage with the new technologies available to our industry.
However, with more sophisticated models – such as digital twins – being created of our dams, it is important to ensure we maintain the guiding engineering principles of verifying input data, validating models for correctness, and building complexity gradually. By doing this, we can provide the necessary assurance and confidence in our increasingly sophisticated and evolving tools.
ABOUT THE AUTHOR
Richard Herweynen is Entura’s Technical Director – Water. He has more than three decades of experience in dam and hydropower engineering, working throughout the Indo-Pacific region on both dam and hydropower projects. His experience covers all aspects including investigations, feasibility studies, detailed design, construction liaison, operation and maintenance and risk assessment for both new and existing projects. Richard has been part of a number of recent expert review panels for major water projects. He participated in the ANCOLD working group for concrete gravity dams and was the Chairman of the ICOLD technical committee on engineering activities in the planning process for water resources projects. Richard has won many engineering excellence and innovation awards (including Engineers Australia’s Professional Engineer of the Year 2012 – Tasmanian Division), and has published more than 30 technical papers on dam engineering.
Decommissioning battery energy storage systems: It’s never too early to plan for retirement
All good things come to an end – even big battery energy storage systems (BESS). In Australia, the end of life for BESS may feel very distant, since we’re really only in the infancy of the booming BESS industry; however, the life expectancy of these big batteries is nothing like the design life of other renewable energy and storage technologies. With BESS currently only lasting for around 10 to 15 years of operation, developers and owners of BESS installations need to be thinking and planning now for what decommissioning will involve and what it will cost. In fact, planning for decommissioning is best done right from the start – long before the project is constructed.
Here, one of Entura’s BESS specialists, Dr Rahmat Khezri, answers some burning questions about the end stages of a BESS project.
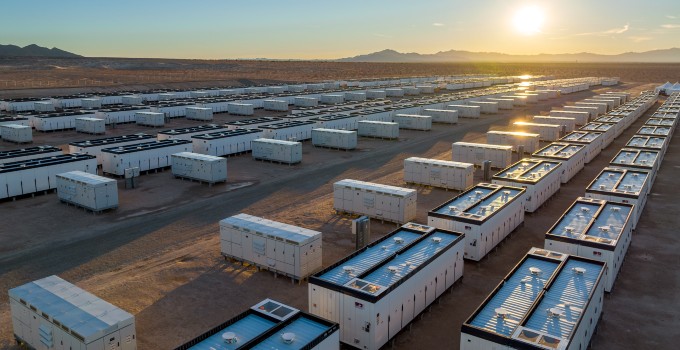
- When does the life of a BESS project come to an end?
The need to decommission a BESS project could arise either from the conclusion of a contract or power purchase agreement – though this is unlikely – or from the expiration of the battery’s operational lifespan. This could be when the battery capacity has degraded to a point where it is no longer financially viable to operate in the market or when the battery’s internal chemistry has reached a point where it is unsafe to continue operating.
For nickel-manganese-cobalt (NMC) batteries, which held a significant share of batteries before lithium-iron-phosphate (LFP) batteries entered the market, this could be after 3,000 to 7,000 cycles at 80% depth of discharge (DoD) or around 10 to 15 years. For LFP batteries, which are gradually dominating the battery market for BESS, it’s around 4,000 to 10,000 cycles or 15 to 20 years. However, several factors can either reduce or extend the battery lifespan. Higher DoD shortens battery life, and frequent high-power cycling (e.g. for frequency control ancillary services, FCAS) can accelerate degradation. Avoiding prolonged high or low state-of-charge management (SoC) reduces degradation. Temperature is also an issue – so proper cooling and heating systems can support greater longevity.
- Is decommissioning the only option?
Full decommissioning of aging BESS assets is not always necessary at battery end of life, as some key components – such as inverters, transformers and balance of plant (PoP) – often retain residual service life. There may be opportunities for repowering or augmentation instead, which will require a much lower level of decommissioning activities. Repowering replaces old batteries with newer or upgraded technology to restore or enhance performance. Augmentation generally aims to increase capacity or power output by expanding the system with additional capacity, for example by adding extra battery racks to an existing setup.
- What does a full BESS decommissioning program involve?
A typical full BESS decommissioning program includes the following activities:
- developing the decommissioning plan including a risk assessment
- de-energising the BESS
- removing power conversion systems (inverter/transformer stations)
- removing integrated battery storage units (site dismantling and packaging may be required)
- shipping the packed battery storage units to recycling facilities
- removing electrical cables and conduits
- removing substation equipment
- shipping the power conversion systems and cables to recyclers of electronic waste
- transporting non-recyclable material removed from the site to disposal facilities
- restoring the site.
- How long could BESS decommissioning take?
Once a BESS project stops operating, the decommissioning process can begin (usually within 12 months) and can take around 6 to 9 months. Of course, this will depend on the scale of the BESS project, which will determine the amount of battery units and electrical infrastructure that will need to be removed from the site. If site restoration is required, this could extend the timeframe.
- What might BESS decommissioning cost?
Decommissioning costs are made up of labour, equipment, transport, materials, and recycling or disposal. These costs can be categorised across the stages of the project: removing the BESS facilities, restoring the site, packaging and transport, and the cost of recycling or disposal. If it is possible to achieve some value from salvage, this can be offset against the decommissioning costs. Currently the total recycling fee for high-powered batteries is around $10–15 per kg. Assuming approximately 6 kg for 1 kWh, the recycling cost would be $60–90 per kWh.
- Talking about recycling, what are the options for decommissioning BESS sustainably?
In Australia, we’re still in the early days of developing a lithium battery recycling industry – but we are seeing increasing recognition by owners, developers, regulators and the community of the need for the renewable energy industry to strengthen the circular economy and minimise landfill. Battery modules are recyclable – but salvage and reuse are likely to remain very dynamic and the future market for used lithium-ion modules remains uncertain. We recommend that every BESS developer/owner should aim to maximise recycling and develop as sustainable a waste management plan as possible. It is worth noting that recycling and reuse of inverters, transformers and BoP components are well established and available.
- What regulatory requirements are there in Australia for BESS decommissioning, and how do you envisage this changing?
In Australia, BESS projects must comply with relevant environmental regulations, which vary by state but generally align with the environmental protection guidelines set by each state’s Environment Protection Authority (EPA). At the federal level, the Product Stewardship Act 2011 promotes the responsible management of products at the end of their life cycle, including batteries. Decommissioned components from BESS projects, particularly lithium-ion batteries, are typically classified as prescribed industrial waste (PIW), requiring handling by licensed transporters and disposal at approved facilities.
At this stage the permitting process to build a BESS facility does not require a formal plan for decommissioning and disposal – but it is probably only a matter of time until we see more stringent requirements as part of the development application process. Despite a detailed plan not yet being required, it is definitely worthwhile doing, not least because the costs of decommissioning should be factored into the business case from the outset.
- What lessons have been learned from international examples of BESS decommissioning?
There haven’t been many examples of BESS decommissioning in our region yet, but the example of decommissioning the Tehachapi Energy Storage Project (TSP) project in South California in 2022 is an instructive real-life case study. This 8 MW / 32 MWh lithium-ion battery project was one of world’s first and largest BESS when it was commissioned in 2014. It had a footprint of 585 m2, with 604 battery racks and two 40-foot PCS containers. The decommissioning was completed in just over 4 months from the generation of the purchase order to the finish.
A key takeaway from this case study is the significant impact that logistical and site-specific constraints can have on project outcomes. Although the PCS containers were initially expected to be removed within a single day, unforeseen space limitations and permitting restrictions ruled out the use of cranes. As a result, the containers had to be dismantled and cut into sections on-site. This increased the schedule by 17% and the cost by 20%. The lesson here is clear: success lies in the details, particularly when it comes to transport and access logistics. Thorough, site-specific planning is essential to avoid unexpected delays and budget overruns.
- What should Australian BESS developers and owners be doing now about decommissioning?
Ideally, every player in the BESS industry in Australia should be thinking ahead, however far off BESS decommissioning, repowering or augmentation may seem right now. It is never too early to start investigating the potential options, costs, timing, emissions, challenges and solutions – and developing a plan that can continue to evolve as the industry matures. A robust decommissioning plan will also be a valuable input into the technical due diligence process for sale/purchase. Ultimately, solid, early planning is the key to minimising risk and maximising value – and achieving a more efficient, more cost-effective and more sustainable outcome for any BESS project.
At Entura, we’ve been involved with BESS since the very start of the sector in Australia, and we’re always learning from our experiences here and around the world. We’re actively developing our analyses of decommissioning costs, transport, timing and circularity opportunities so that we can support your BESS project holistically, from cradle to grave.
Regardless of the stage of your BESS project, Entura can help. Contact Patrick Pease to find out how.
About the author
Senior Renewable Energy and BESS Engineer Dr Rahmat Khezri has vast professional and technical experience with batteries. He has worked in the renewable energy and battery industry in project delivery from BESS design, business case and feasibility analysis to operation and construction. Rahmat has managed several utility-scale BESS projects during his time with Entura, overseeing successful delivery while ensuring compliance with industry standards, optimising performance, and managing key stakeholder relationships. Before joining Entura, he worked on projects supported by Sustainability Victoria for technical design and business case development of ‘second-life BESS’ using retired batteries of electric vehicles. In 2023 and 2024, he was recognised by Stanford University as being in the top 2% of scientists worldwide for two consecutive years.
What have we learnt about renewable microgrids and remote area power systems?
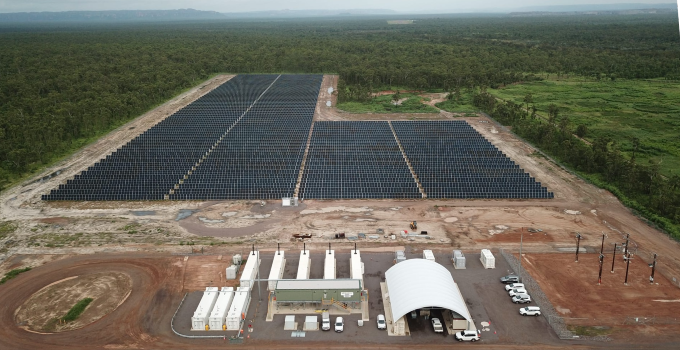
With the evolution of modern inverter and renewable energy technologies, it’s become possible to build microgrids with very high renewable penetration. These renewable solutions are revolutionising electricity sustainability, reliability and access in many far-flung locations – such as Pacific islands, remote locations around Australia, and on mining sites.
High renewable penetration allows operators of off-grid microgrids to extract maximum value from their installed solar, wind or other renewable generation while minimising the use of fossil fuels, such as diesel. It’s a win-win for the financial bottom line and emissions-reduction goals. If the microgrid connects with a broader power system, the benefits of reducing reliance on externally sourced energy are significant too.
Establishing a relatively simple microgrid with modest fuel-saving targets doesn’t have to be particularly complex. It’s within the reach of current technology and practice to be able to optimise microgrids to all but eliminate the use of fossil fuel (with the related advantages of eliminating fuel handling, shipping, etc.). However, careful thought must be given to the distinct nature of the microgrid: its customers, energy sources and storage options.
To make a microgrid successful, some technical challenges will need to be managed. Resolving these challenges in a cost-effective way becomes more difficult as the renewable energy balance approaches 100% – but they’re not insurmountable. Let’s explore.
Challenge 1: Ensuring quality of supply and an acceptable customer experience
The small size of microgrids and the fact that they are usually dedicated to a single ‘customer’ makes engineering a microgrid a sensitive task. While larger grids can operate with tight voltage and frequency tolerances and multiple levels of redundancy, microgrids often cannot. This inevitably means negotiating acceptable system standards for islanded operation that are within the tolerance of customers’ equipment and expectations. Microgrids powered by fossil fuels typically have more difficulty maintaining tight frequency and voltage tolerances than renewables-based microgrids.
Understanding customer and stakeholder expectations relating to reliability and robustness (energy availability and the ability to ride-through faults) is key to the establishment of a successful microgrid. Where the microgrid exists with no possibility of interconnection to another power network (i.e. in an ‘island’), there is more flexibility and less operational complexity. Discerning acceptable standards and practices is still not easy but it can be a more productive discussion than when the microgrid must comply with less tailored standards due to interconnection.
When the microgrid is interconnected, many of the technical requirements for the microgrid will be specified and/or mandated by the network service provider, electricity code requirements, and some operational requirements for electrical safety. If additional communication and inter-tripping with network equipment remote to the microgrid are required, these can add further complexity.
Challenge 2: Managing different modes of operation requiring different controls
In the previous section, we discussed how complicated it is to determine system standards. A great deal of care is also needed when contemplating which standards and controls are most appropriate to the microgrid’s specific characteristics. This is where cookie-cutter solutions could under-deliver and a more insightful approach is required.
A microgrid must manage the voltage, frequency and quality of supply while it is islanded as well as during periods of interconnection. Islands can remain satisfactory for longer if there are energy sources, storage and load controls within the island. Each of these elements comes at a cost and, depending on the frequency and duration of islanded operation, must have a value outside of the islanded scenario.
Our experience with microgrids is that semi-autonomous operation of each of the power sources using standard power system control approaches (solar, BESS and other power sources on voltage and frequency droop control) leads to the simplest and most secure response to transients (load or generation trips, network or network faults). Slower controls can be put in place to balance generation and rates of battery charge or discharge. If the battery power rating is large enough relative to the largest disturbance, it can manage most frequency disturbances within acceptable limits. If the battery energy capacity is large enough, it can always maintain a state of charge that allows fast reaction to variations in customer demand or VRE. Alternatively, auxiliary plant such as switched resistors, synchronous condensers and customer-level load control can be used to minimise battery power, storage and other energy inputs.
Operating microgrids in different modes requires careful engineering of the controls and equipment. The solutions and approaches described above have proven successful across multiple projects, but the key is to always be open to new solutions as new problems arise or new technologies emerge.
Challenge 3: Understanding the marginal value of resistors, synchronous condensers and/or demand management
Auxiliary equipment can help to extend the range and effectiveness of a microgrid to rely solely on renewable energy. The challenge is to understand the actual value of extension in this context. Where it has a direct bearing on fossil fuel use, the benefits are clear. Where it might cause an incremental improvement in the robustness of the islanded operation only, it’s important to consider whether any operational benefits or security benefits gained from the additional capital investment are valuable enough to justify the cost.
If the robustness of islanded operation is to be increased by managing customer demand, customer storage and/or embedded generation (e.g. rooftop solar), the value of the extended island longevity should be weighed against the perceived cost (direct or indirect) to the customer. This requires careful consideration of the diversity within the community, their openness to energy management, and the relationship between true costs and benefits.
Microgrids that maximise local use of renewable resources represent a relatively cost-effective option for lowering carbon emissions and/or reducing energy costs. Isolated communities, remote industrial sites, unreliably connected rural communities and others could benefit greatly from considering microgrid opportunities.
Entura has a long history of design, formation and operation of microgrids throughout Australia (such as King Island, Flinders Island, Rottnest Island, and at mining sites such as the Agnew gold mine) and in the Pacific region (including the Cook Islands, the Federated States of Micronesia, Tonga, and the Solomon Islands, to name just a few). We’ve seen the real benefits these systems bring to our clients and communities.
Contact us if you’re interested in unlocking the full potential of microgrids for your operation or community, or if you’re interested in ways to increase your use of renewable energy.
ABOUT THE AUTHORS
Donald Vaughan has over 20 years’ experience providing advice on regulatory and technical requirements for generators, substations and transmission systems. He has worked for all areas of the electrical industry, including generators, equipment suppliers, customers, NSPs and market operators. Donald specialises in the performance of power systems. His experience in generating units, governors and excitation systems provides a helpful perspective on how the physical electrical network behaves.
Greg Koppens is Entura’s Principal Control Engineer Hybrid Renewable Systems and has led Entura’s secondary electrical engineering team. Greg’s experience spans power, oil and gas, and mining, including onsite roles. With over a decade of detailed design experience, Greg facilitates collaboration between engineering disciplines and other stakeholders to solve complex problems. He regularly shares his extensive expertise with the mining sector to advance their decarbonisation goals. Find out more about Greg in our podcast series here.
We built this synchronicity … but what now?
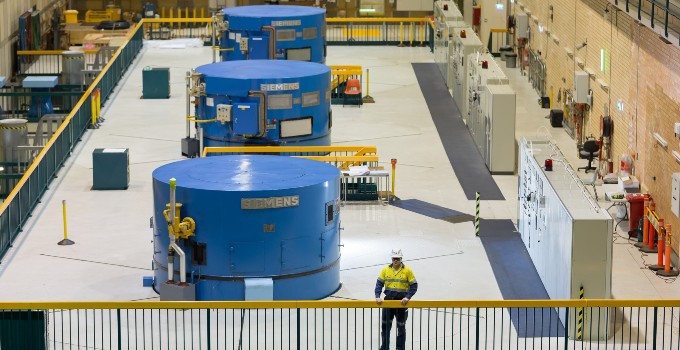
The alternating current (AC) transmission age started in 1891 through collaboration and perseverance. This transmission method has transformed the world, led us to the brink (or over the brink) of a climate disaster, and provides the backbone for a future founded on abundant renewable resources (in Australia, at least). Collaboration and perseverance are now required to ensure the continued utility of the grid.
Synchronous machines have always been the driving force in the grid. These elegantly simple electrical machines sit in powerhouses around the globe using their century-old technology (nearly 150 years, in fact) to convert mechanical energy into electrical energy to power the computer that I’m using to type this.
There’s a romance to these machines. It’s more than nostalgia. They spin, as the name suggests, in synchronism. If one of them falters, all the rest pick up the slack. The only connection they need is the power lines. This robustness is central to the way our electricity grids have operated for over 130 years – but the influence of synchronous machines is slowly being eroded.
The most obvious erosion of the influence of synchronous machines is their displacement by inverter-based renewable (IBR) technologies as solar, wind and battery energy storage (BESS) installations proliferate. A less obvious erosion of their influence is the diminishing understanding and appreciation of synchronous technology within the industry. This lack of understanding by engineers, planners and regulators of the fundamental building blocks of the electricity grid is starting to show.
This might sound like the curmudgeonly ranting of yesterday’s engineer as technology passes them by. Maybe it is … but, rather than dwell on negatives, let’s look at what synchronous machines bring to the power system.
- System strength
The fundamental difference between synchronous machines and IBR is thermal inertia. Typical synchronous machine design can sustain high levels of over-current for a relatively long time (seconds) compared to IBR units (milliseconds). This difference allows synchronous machines to provide a strong ‘natural’ response to voltage variations in the power system without threat of overload and damage. Transmission protection systems – and therefore grid security and safety – rely on this characteristic.
There are alternatives. Overload capacity can be built into inverters, but this is expensive. Dedicated inverter-based devices such as static synchronous compensators (STATCOMs) can be used to provide fault response.
- Inertia
Synchronous machines spin. Their spinning bits (rotors) have mass, so they have mechanical inertia. This mechanical inertia doesn’t require a control system to provide an inertial response. The inertial response from a synchronous machine is a known, predictable quantity, regardless of voltage.
IBRs can provide synthetic inertia and, when voltage is healthy, can out-perform synchronous units. When voltage is not nominal, the same current limitations that affect the IBRs’ ability to deliver fault level can also restrict the effectiveness of synthetic inertia delivery.
- Robustness
The previous two characteristics – system strength and inertia – show the support that synchronous machines can provide to the power system during disturbances. The synchronous machine can deliver these supports across a wide range of power system disturbances to voltage and frequency.
IBRs typically rely on fast controls to manage their response to system disturbances. Under some extreme conditions these controls may not be fast enough or well enough tuned to manage. While tuning is important to synchronous machine performance, often it has a second-order effect or adds robustness over and above the natural response.
Displaced but not superseded
Understanding the inherent dynamics of synchronous machines gives power system engineers a better appreciation of these machines’ contribution to power system stability. Regulators should be mindful of the reduced risk (and mostly advantages) to system security that synchronous machines offer relative to IBRs. This is despite the uncontrollable nature of synchronous machines. That is, physics dictates the stabilising effects from synchronous machines whereas control algorithms determine whether an IBR can stabilise or destabilise. Controller model accuracy is therefore more important for IBRs than it is for synchronous machines where transient stability or electro-mechanical transient time frames are considered.
Better understanding of synchronous machines should lead to more appropriate rules relating to dynamic response to system events. Overly specific requirements for fault ride-through, power recovery post-fault, and maintenance of real and reactive power during voltage disturbances may all lead to needless protracted negotiations over access standards. This slows down the progress of the energy transition, frustrates otherwise helpful development, and diverts resources towards trivial considerations rather than focusing on issues of greater importance.
Synchronous machines will play a role in the energy grid of the future. Our industry needs to maintain expertise and regulatory frameworks that allow this technology to continue providing the grid with vital stabilisation and robustness.
ABOUT THE AUTHOR
Donald has over 20 years’ experience providing advice on regulatory and technical requirements for generators, substations and transmission systems. He has worked for all areas of the electrical industry, including generators, equipment suppliers, customers, NSPs and market operators. Donald specialises in the performance of power systems. His experience in generating units, governors and excitation systems provides a helpful perspective on how the physical electrical network behaves.
Understanding the business risks of small dams and weirs
Small dams may pose significant business risks that are often under-appreciated, even if these dams don’t pose a safety risk to the community. Managing risk is a key part of running any sustainable business and understanding how to mitigate risks requires that they are properly identified, analysed and evaluated.
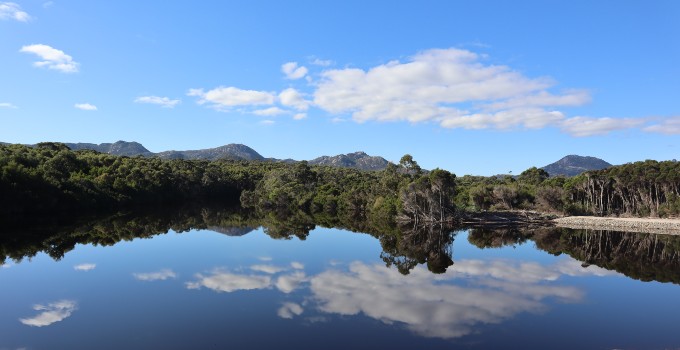
The Guidelines on Risk Assessment prepared by ANCOLD (Australian National Committee on Large Dams) provides a detailed process for quantitative analysis of dam safety risks for large high-consequence dams, but adopting this process for small dams and weirs can be costly and may not be clearly justifiable.
For owners of small dams, ANCOLD has a number of other guidelines that can be useful for managing these dams, including Guidelines on the Consequence Categories for Dams and Guidelines on Dam Safety Management. Assigning a consequence category for a small dam can be a useful first step in understanding the risks – and will consider the impacts on community safety, on the environment, on the dam owner’s business, and on other social factors including impacts on health, community and business dislocation, loss of employment and damage to recreational facilities and heritage.
The consequence categories are graded from ‘Low’ to ‘Extreme’. These categories are used for a number of purposes including:
- regulatory requirements (depending on which state the dam is in)
- recommended surveillance and monitoring activities
- maintenance and operational requirements
- spillway flood capacity
- dam design standards.
The focus of ANCOLD’s consequence category guidelines is on wider community safety and impacts, but not on the dam owner’s business. This potentially leaves the dam owner exposed to significant unidentified business risks. Ideally, these should be managed consistently alongside all the other business risks.
A structured approach to assessing the business risks of small dams
ANCOLD’s Guidelines on Risk Assessment is a useful starting point for undertaking a business-focused risk assessment of small dam assets. As with all risk assessments, it is useful to follow a structured approach, including the following steps:
- identify the hazards
- brainstorm the failure modes
- estimate the likelihood of the failure
- estimate the consequences of failure
- evaluate the risks
- develop risk mitigation measures.
Such a risk assessment approach is ideally completed with a dam engineer working closely with the business owner to capture both the dam engineering and the business-specific knowledge.
1. Hazards
Dams need to be properly designed, constructed and maintained to continue to perform their function safely. It is essential to avoid becoming complacent. Floods are a significant hazard to all dams and cause around 50% of all failures in large, well-engineered embankment dams. Small dams are often constructed with no or minimal engineering input into the design or construction and as a result may have inherent defects that may not manifest themselves until years later.
Dams in general do not require a lot of maintenance; however, a lack of suitable maintenance can lead to failures. A key maintenance activity is management of vegetation so that trees do not establish themselves in the embankment. Tree roots can create leakage paths that could lead to piping or internal erosion, and ultimately to a failure.
2. Failure modes
A key part of the expertise of a dams engineer is understanding how different types of dams can fail, which is crucial for identifying potential failure modes. The ANCOLD guidelines on risk assessment recommend completing a site inspection of the dam to help identify the key ways in which the dam could fail. The inspection should be conducted with the dam owner to look for evidence of failure modes, such as:
- deformation or cracking, which may indicate issues with the stability of the dam
- wet areas or flows through the dam, which may indicate a piping failure
- spillways where the original crest is filled in or raised to increase storage in the reservoir, which can often be an area of concern
- erosion close to the dam from operation of the spillway, which could lead to undermining and instability of the dam wall.
Typically, failure modes are identified in a workshop setting and then prioritised by criticality. The full list of failure modes is then reduced to a shortlist of those that are most critical.
3. Likelihood of failure
ANCOLD’s Guidelines on Risk Assessment provides an approach that can be used for detailed quantitative risk assessments; however, such approaches require significant effort to apply and can be costly. For small dams, it can be more appropriate to use a risk matrix approach, similar to that outlined in the Australian standard AS ISO 31000 Risk Management.
Typically, most businesses have a standard risk assessment procedure that can be adapted to give a qualitative or semi-qualitative assessment of likelihood. An experienced dams engineer will be able to assign a likelihood for each of the credible failure modes based on engineering judgement and some simple calculations (e.g. using regional flood estimates and estimates of the spillway discharge capacity). Failure modes for dams that are well designed and constructed will often have a likelihood rating of ‘Rare’ or ‘Unlikely’. The likelihood may be higher for dams in poor condition or with identified deficiencies.
4. Consequences of failure
A business risk assessment focuses on the consequences to the business, rather than the wider community, if the small dam were to fail. This will be unique to each business and will need input from the owner. It can be assessed by working through a series of questions about the need for the dam and its purpose – for example:
- What is the water in the dam used for? Can the business function without the water or the storage space in the dam?
- Are there alternative sources for the water that can be quickly accessed, and will these be sufficient for normal operations or would it be necessary to reduce operation?
- Is there business infrastructure downstream of the dam, and could a failure of the dam cause failure of these assets (e.g. pumping stations, water treatment plants or other dams) that would impact business operations? Can the business operate without these assets?
- How will customers be affected and what are the reputational consequences of not being able to supply or only partially supply?
- What are the financial implications for the business, and is there insurance that would cover the cost of the event, including consequential losses?
- How long would it take to replace the dam (including refilling) and the other assets?
5. Evaluation of risks
Using the business’s standard risk assessment tool enables comparison of the small dam risks against other business risks on a consistent basis (e.g. safety risks to employees). The level of risk will indicate the urgency of addressing the risk. This process allows a clearly articulated justification to be presented to the business for putting in place any required mitigations. It also enables the owner to focus on the key business risks rather than become distracted by issues with lower risk.
6. Risk mitigation
Mitigations can address either likelihood or consequences and will need to be tailored to the specific risks and the business needs. Addressing the risks by reducing the likelihood will typically involve physical works to the dam – for example, increasing the size of the spillway to reduce the likelihood of an overtopping failure, or managing vegetation to reduce the likelihood of a piping failure.
Where reducing the likelihood is not practical or not sufficient, addressing the consequences may be an effective approach. Addressing the consequences may involve options such as securing alternative water supplies, contingency planning to reduce impacts on customers, or insurance to cover the financial losses.
Bringing it all together for better business insights
Entura has undertaken qualitative and semi-qualitative small dam risk assessments for a number of clients in a cooperative environment to bring together our dams engineering expertise with the owner’s knowledge of their business. This is a cost-effective approach that has provided clarity on the specific business risks related to small dams, allowing targeted risk mitigation measures to be put in place. The process has provided important insights enabling owners to justify business decisions and reduce their overall business risk exposure.
If you have small dams and would like to talk with us about assessing your business risks, contact Phillip Ellerton or Richard Herweynen.
About the author
Paul Southcott is Entura’s Senior Principal – Dams and Headworks. Paul has an outstanding depth of knowledge and skill developed over more than 3 decades in the fields of civil and dam engineering. He is a highly respected dams specialist and was recognised as Tasmania’s Professional Engineer of the Year in Engineers Australia’s 2021 Engineering Excellence Awards. Paul has contributed to many major dam and hydropower projects in Australia and abroad, including Tasmania’s ‘Battery of the Nation’, the Tarraleah hydropower scheme, Snowy Hydro, and numerous programs of work for water utilities including SeqWater, Sun Water and SAWater. His expertise is a crucial part of Entura’s ongoing support for upgrade and safety works for Hydro Tasmania’s and TasWater’s extensive dams portfolios. Paul is passionate about furthering the engineering profession through knowledge sharing, and has supported many young and emerging engineers through training and mentoring.
The life cycle of a dam – Bringing it all together
Dams, like all of us, go through several life stages. Some dams have harder lives. Some age more quickly. Some need a lot of attention, and some are more robust. Let’s talk a bit about a dam’s life – and revisit some of our previous articles on dam engineering.
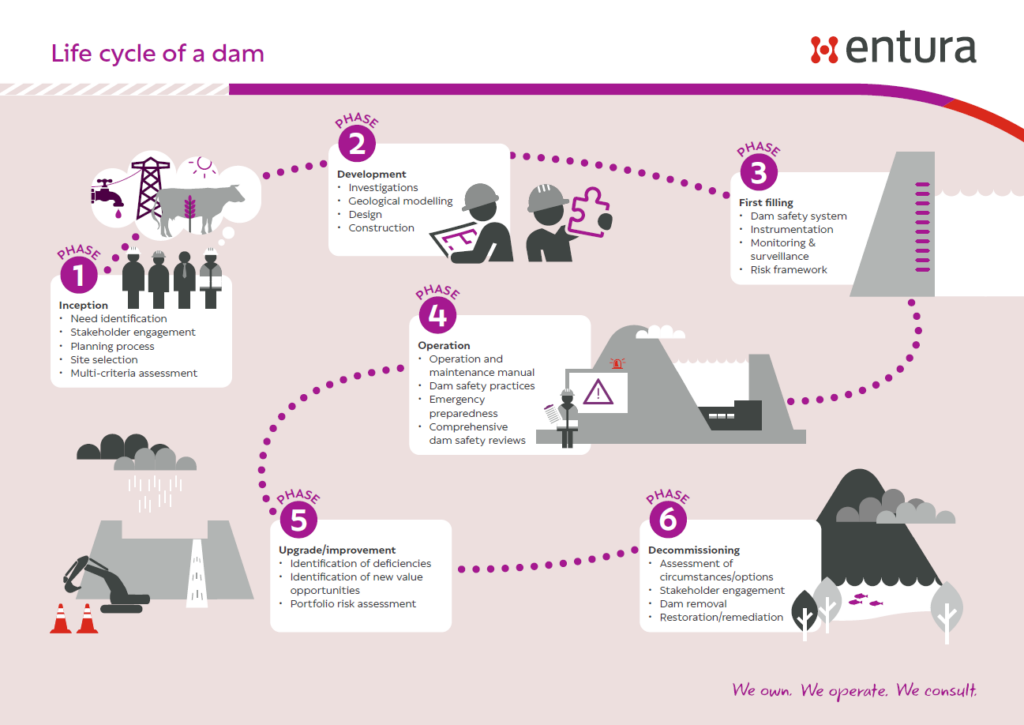
Phase 1: Inception
The starting point of the dam life cycle is the planning process – where a need is identified and it is determined that the way to meet that need is to create a water storage by constructing a dam. It is essential that this planning process involves effective stakeholder engagement. Although there may be a primary purpose for the dam, it is very common through the stakeholder engagement process to consider other benefits that the dam could provide, making it a multipurpose dam.
The planning process will lead to the site selection stage. Choosing a suitable site which is both technically sound and environmentally and socially acceptable will have a significant impact on the remaining stages of the dam’s life. Multi-criteria assessment can help get the selection right, ensuring technical, financial, environmental and social aspects are considered in a balanced way.
Read our articles:
- ‘No dams’ or ‘right dams’? That is the question
- Make better decisions about hydropower and dam project options using risk-based multi-criteria assessment
Phase 2: Development
The development phase includes the investigation, design and construction of the dam. Every dam site is different, and it is important to understand this. As a result, the ideal dam type for one location will not be the same as for another location.
Read our article:
It is important that the risks associated with the dam site are known and understood. A key risk is the geological aspects of the dam’s foundation. Are there defects that could impact the stability of the dam? Are the foundations erodible? Could permeability be an issue? A staged investigation program formulated around the geological model will help to provide this understanding.
Read our article:
Design must be in accordance with current practice, guided by engineering standards and guidelines such as ANCOLD guidelines and ICOLD bulletins. Construction needs to be in accordance with the design and should be conducted using an appropriate quality assurance system and quality control program. An Independent Technical Review Panel (ITRP) helps avoid anything falling through the cracks. (The Queensland Dam Safety Management Guideline provides some guidance about this.) An ITRP will provide strong technical governance during design and construction, utilising the collective knowledge and experience of its members.
Read our article:
Phase 3: First filling
The next phase of the dam’s life is the first filling. This is a very exciting time, but it is also known to be the highest risk stage of a dam’s life. As a result, we need to be prepared. A dam safety system needs to be in place, along with the necessary instrumentation to monitor the dam during this first fill.
Read our article:
In case of any incident occurring during first filling, it’s crucial that the dam safety emergency plan has been prepared and the dam safety manager identified. As the dam fills, there should be a heightened level of monitoring and surveillance, using this information to compare the actual performance against what was expected.
Entura has used a risk framework to determine a dam’s readiness to impound, such as for Murum Dam in Malaysia. Of course, some reservoirs take a long time to fill, potentially over a number of years, so this heightened level of monitoring and surveillance could go on for some time. There could also be saddle dams that experience water against them much later than the main dam.
Phase 4: Operation
Now begins what, hopefully, will be a long phase of normal operation. The dam will have an operation and maintenance manual to ensure that the dam is operated as intended and regular routines occur. Good dam safety practices must continue throughout the operational life, including dam surveillance, routine inspections, and ongoing emergency preparedness should any dam safety incidents, major floods or seismic events occur. Emergency plans should be tested regularly to ensure they are appropriate and robust.
Read our articles:
- What can dam owners do to better manage floods and avoid the blame game?
- How robust is your emergency preparedness?
During the operational phase of a dam, it is also important that comprehensive dam safety reviews (DSRs) occur every 20 years, or whenever there has been a major event or a change in standards or guidelines. The intent of a DSR is to determine the safety of the dam against current practice and the current condition of the dam. It’s important that the DSR considers the potential failure modes for the dam.
To undertake a DSR, good historical documentation for the dam will be needed. If the records aren’t great, or there are significant gaps, the DSR may require additional investigations and analysis to be undertaken.
Read our article:
In addition, it is critical that the public is kept safe around dams and throughout the operation of dams. In 2012 ICOLD established a working committee to identify these public safety risks, describe the international state of practice to manage and mitigate the risks, and develop a guidance bulletin on best-practice measures and public education about safety around dams.
Read our article:
Phase 5: Upgrade and improvement
If the DSR identifies deficiencies in the dam, a dam safety upgrade may be needed. This is the next stage of a dam’s life. A risk framework can often be used to justify and guide these upgrades.
Read our article:
Dam upgrades may not always be due to a dam safety issue; they may also be driven by the opportunity to increase value, which may be able to be achieved through measures such as raising the height of the dam. They can also be driven by changing design standards, changes to legislation, greater understanding about extreme hazards, or (more recently) climate change impacts.
Read our article:
With a large portfolio of dams, the demand on resources (both capital and human) can be significant. A portfolio risk assessment (PRA) allows owners of dams and other water assets to see the bigger picture of how to prioritise their efforts and resources to achieve the best safety results across the whole portfolio.
Read our articles:
- Portfolio risk assessment takes dam safety programs to the next level
- Safer dams are a matter of priority
Phase 6: Decommissioning
This final phase of a dam’s life may actually never occur, as most dams continue to provide a valuable service to society indefinitely. But, with time, the needs of the community may change, or the commercial benefits of the dam may reduce. In these circumstances, the dam may be decommissioned and removed. This decision is not likely to be made quickly, and for good reason, as this is a very complex matter involving many stakeholders. A recent example is the landmark decision to remove 4 dams along the Klamath River in northern California and southern Oregon. This is the most extensive dam removal and river restoration project in US history.
Although some dams may at some stage be decommissioned and removed, more dams will always be needed to meet the world’s needs for water security, clean energy, and storage of mining tailings.
Read our article:
And so the life cycle begins, all over again.
(more…)Getting the answers you need: Technical due diligence for vendors, buyers, lenders or insurers of renewable energy projects
Technical due diligence (TDD) for a renewable energy project evaluates the risks and opportunities associated with the project to establish its technical and financial viability, compliance with regulations, and other factors influencing its value. TDD is fundamentally a well-understood risk assessment process, with practitioners typically having templates they can tailor to specific projects and client needs. However, different parties will have different objectives, different scope for TDD to cover, and different risk appetites.
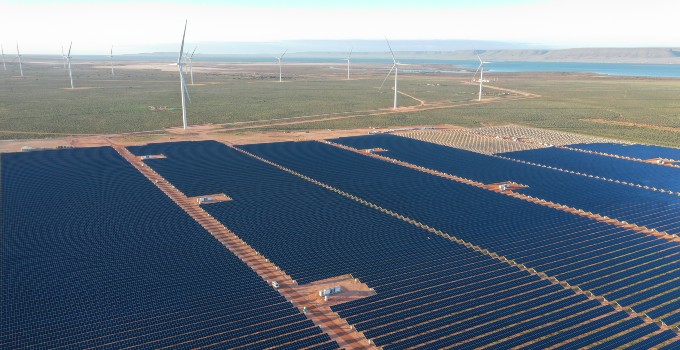
Objectives
Vendor perspective: Do we have our house in order?
An owner (or vendor) is most likely to procure TDD to show the market and prospective buyers their project or portfolio of assets for sale. Engaging an ‘independent’ party to prepare a TDD report serves several purposes: it can facilitate a quicker sale process, enable the owner to anticipate a buyer’s questions, and allow the owner to take pre-emptive actions to mitigate perceived risks. This can give the owner greater control over the sale process or how the project is later structured. For example, in Australia, there is a trend of wind turbine suppliers (original equipment manufacturers, OEMs) getting involved early and developing the project around their specific wind turbine model, then selling the project while maintaining a strong interest as the OEM.
Buyer perspective: Is this project worth our investment?
A buyer conducts TDD to evaluate whether the project aligns with their investment goals, operational capabilities, and risk tolerance. They are interested in understanding the technical feasibility, potential challenges, and opportunities associated with the project. There is often a need for technical inputs to feed into a financial business case, such as CAPEX and OPEX estimates and estimates of energy yield.
Lender perspective: Will we get our money back?
Lenders, such as banks or funding agencies (e.g. Clean Energy Corporation, ARENA, World Bank, Asian Development Bank), conduct TDD to assess the project’s ‘bankability’. This involves evaluating whether the developer can fund the project and if the project can generate sufficient returns to repay loans. Lenders focus on mitigating financial risks and ensuring compliance with lending criteria and industry standards.
Insurer perspective: Is this project insurable, and at what cost?
A wide range of insurances may be in place during the construction and operation of a renewable energy project. Insurers will evaluate project risks and feasibility to determine insurability, the cost to insure, and how to manage potential claims effectively.
Scope
Vendor perspective: Help us prove to buyers that we’re transparent
A prudent vendor will try to anticipate everything buyers might want to know and present the project in the best possible light, ideally addressing information gaps and mitigating risks. The vendor will rely on the consultant’s independence for the TDD report to have value for potential buyers.
Buyer perspective: Tell us the risks – and if the project can be improved
A buyer may undertake TDD at any stage of the project life cycle, from conception to decommissioning, requiring a tailored scope of work. The buyer will focus on technical aspects impacting ownership and operational responsibilities, such as technology selection, construction feasibility, resource assessment, and operational performance expectations. Existing assets may also influence their priorities during TDD.
For example, a buyer who already operates a portfolio of wind farms will be much more comfortable with many of the typical risks of wind development in comparison to a buyer for whom the project is a first of its kind.
Owners will often employ a consulting business to undertake TDD alongside environmental, social and governance (ESG) due diligence, with legal and financial due diligence conducted by other specialist parties.
A good TDD for an owner will focus not only on risks, but also on opportunities for improving the project.
Lender perspective: Tell us everything!
Lenders sometimes directly engage a consultant for TDD; however, often the owner will manage the engagement of a ‘lender’s’ or ‘independent’ engineer for TDD, with the resulting TDD report provided to a bank or consortium of banks for their evaluation and questioning.
Lender’s TDD engagements typically have a broad scope, encompassing not only technical aspects but also financial, legal and regulatory considerations. They assess the project’s financial model, revenue projections, contractual agreements, insurance, permitting, and compliance with regulatory requirements. Lenders are interested in the capability and experience of key contractors, the robustness of contractual arrangements, and will look to TDD consultants for assurances that project schedules are achievable.
Lenders will have a particular interest in things like budget contingencies, and what contractual mechanisms offer assurances such as warranties and liquidated damages.
In practice, the TDD scope for a ‘shovel-ready’ project may be similar for a buyer and for a lender considering investment.
Insurer perspective: Is the technology mature, and what’s this we’ve heard about a flood zone?
Insurers assess risks, including vulnerabilities, operational disruptions, and compliance with industry standards. They are particularly interested in technology maturity and environmental risks that may lead to future insurance claims. For example, rapidly released new wind turbine models may increase the risk of failures, making insurers keenly interested in operational hours as a measure of a model’s proven reliability.
TDD is a core activity for insurance underwriters (those who evaluate and analyse the risk) and is not typically outsourced to consultants. But consultants can learn from the insurance industry, which deals in risk assessment every day.
Risk appetite
Vendor perspective: Help us show it’s a safe bet
Naturally, vendors will typically prefer to present risks as identified and under control. However, it is in their interest to present the project honestly. After all, many transactions have the vendor retaining a stake in the project.
Buyer perspective: Help us find a project with low risk and good returns
The buyer may be evaluating technical risks during project development, construction or long-term operation depending on the project status. In practice, the earlier in the project’s life cycle, the greater the risk, and, in theory, the greater potential financial return.
For example, a project claiming to be ‘shovel-ready’ should have all major planning permits in place, a confirmed grid connection, and technical studies completed (such as geotechnical investigations). The TDD assessment will review documentation to identify specific risks that might eventuate during construction and operation.
On the other hand, for a project in early development, the risks may be of a more generic nature and the buyer may need to accept that there are some unknowns. This is essentially greater risk but there is potential for greater rewards in the long run.
Lender perspective: We’re taking a lot of risk here, so has everyone done their homework?
A typical funding model is where a bank or syndicate of banks provides a loan to a project owner that is subsequently paid back via the revenue earned by the project. The loan is secured against the assets of the project, and the banks want to ensure they will be repaid. Lenders take on substantial risk; hence, during TDD they expect to see that projects are well-conceived and executed, and they will adopt relatively conservative views on issues such as expected energy yield.
Insurer perspective: What’s the right level/cost of cover?
Insurers have sophisticated risk modelling techniques and real-world experience of what is going wrong on projects and what it’s costing. Insurance is a global industry, and what happens overseas impacts the TDD that insurers do for Australian projects.
Decisions made by project owners can have a substantial impact on the cost of insurance, and in fact whether insurance is even obtainable. For example, if an owner selects a relatively new or untested wind turbine model, the cost of insurance may negate any intended savings.
In summary
While vendors, buyers, lenders and insurers conduct TDD to assess the feasibility and risks of a renewable energy project, their perspectives, objectives, scopes and decision-making criteria differ based on their roles and interests in the project. As technical consultants who conduct TDD, it’s our responsibility to understand these differences and tailor our TDD approach accordingly.
If you’d like to talk with our specialists about technical due diligence for a renewable energy project, contact Patrick Pease, Bunfu Yu or Shekhar Prince.
About the authors
Andrew Wright is Entura’s Senior Principal, Renewables and Energy Storage. He has more than 20 years of experience in the renewable energy sector spanning resource assessment, site identification, equipment selection (wind and solar), development of technical documentation and contractual agreements, operational assessments and owner’s/lender’s engineering services. Andrew has worked closely with Entura’s key clients and wind farm operators on operational projects, including analysing wind turbine performance data to identify reasons for wind farm underperformance and for estimates of long-term energy output. He has an in-depth understanding of the energy industry in Australia, while his international consulting experience includes New Zealand, China, India, Bhutan, Sri Lanka, the Philippines and Micronesia.
Brendon Bateman is a senior renewable energy engineer with more than 20 years of experience on renewable energy projects in Australia, New Zealand, Pacific islands, Philippines, China, India, Sri Lanka, North Korea and South Africa. Brendon is recognised as a technical expert for feasibility or due diligence of renewable energy projects with involvement in the assessment of over 10,000 MW of greenfield and operational projects, identifying key risks for developers, banks, equity funds and aid agencies looking to develop or invest in renewable energy projects. Brendon is highly experienced in the areas of resource assessment, energy estimates and their associated uncertainty, operational performance assessments, wind turbine due diligence and O&M practices.
From binoculars and boots to bytes and bots: harnessing remote sensing and AI for ecological monitoring
For power and water developments to be truly sustainable, we must preserve and protect biodiversity. But it can be difficult to look after what you don’t know about or don’t understand. In the age of Big Data, automation, AI and increasingly clever gadgets, field ecologists can now do more with less – in other words, get lots of good information very quickly and with far less cost. That’s good for projects and for our planet.
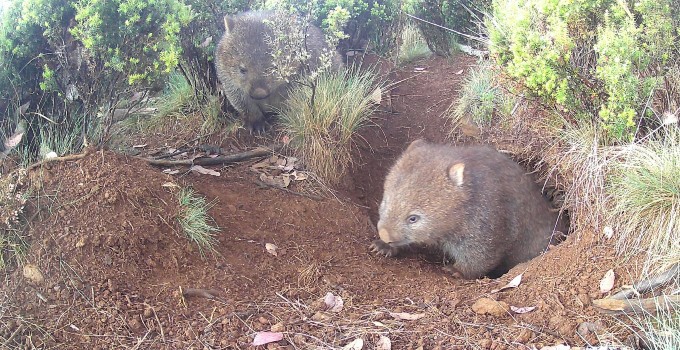
Field ecologists spend much of our time gathering information on species occurrence, distribution, abundance, habitat requirements, and threats. We need methods to detect and quantify biodiversity that are efficient and sensitive, and not biased, invasive or destructive.
Our job increasingly involves leveraging the advances in monitoring technology, computing power, and machine-learning methods to help our clients assess, avoid, mitigate or offset environmental impacts. Vast amounts of visual, spatial, genetic and acoustic information can now be captured using new tools such as ‘camera traps’, automated image classifiers, passive acoustic monitoring, automated species detection from audio data, and eDNA.
Camera trapping
Camera trap monitoring (using digital cameras activated by motion or heat) is a powerful tool for observing and cataloguing species, but it can generate enormous numbers of images. Each image needs to be viewed and tagged to create meaningful data. Until now, that’s taken up a lot of human time. Now, however, machine-learning models can automate the process of detecting and classifying animals.
For example, the ‘MegaDetector’ is an open-source image-segmentation tool from Google that can automatically place a bounding box around a region of interest in the environment, in this case zooming in on an animal and isolating it from the background. This can be put into a wildlife-species classifier before verification by a human, raising the accuracy of classifying some species to up to 99% and increasing the speed at least 20-fold – in fact, it is estimated that approximately 5,000 images can be tagged per hour using these workflows.
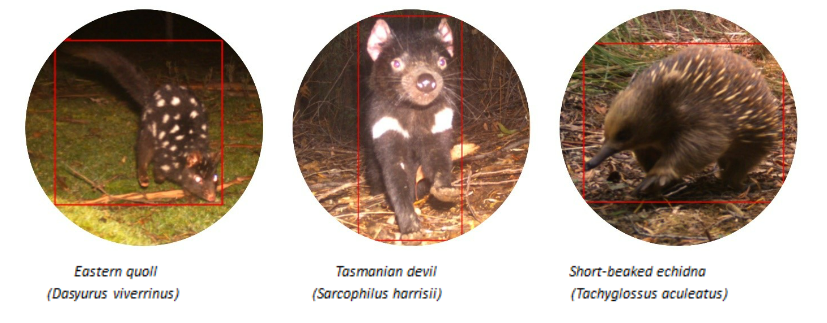
Examples of camera trap images with the MegaDetector bounding box applied
As well as detecting rare, cryptic and elusive native species, camera trapping can also detect and help to quantify the threat posed by introduced animals. Technology has even been developed that enables humane, automated feral cat and fox control: the ‘Felixer’ device uses rangefinder sensors to distinguish target cats and foxes from non-target wildlife and humans. Felixers can even be programmed to play a variety of audio lures to attract feral cats and foxes. The targets are detected via a camera-based AI system working in tandem with four LiDARs. These devices are operating in all Australian states and territories, protecting threatened species including bilbies, bettongs, rock wallabies, quolls, malleefowl, ground parrots, numbats and rare dunnarts and rodents.
Passive acoustic monitoring
Another recent advance that is revolutionising species detection is passive acoustic monitoring. In Tasmania, the endangered, cryptic, poorly understood Tasmanian masked owl (Tyto novaehollandiae castanops) has traditionally been detected through ‘call-playback surveys’ – experts listening for owl vocalisations in response to broadcasting recorded owl calls – but some owls just won’t play the game! Passive acoustic monitoring is a more effective method for detecting these birds, with recorders deployed and set to record from dusk until dawn. Software has been developed to graph the recorded sounds as spectrograms and then automatically detect this species’ persistent screech calls and even chattering calls. Work is underway to differentiate between adult and juvenile calls, which will help identify nearby roosting and nesting sites. With robust bioacoustic recorders and partial automation of analysis, we can detect this elusive species and identify critically important nesting sites more accurately, rapidly and at less cost. The technology can also be used to detect other species with distinctive vocalisations.
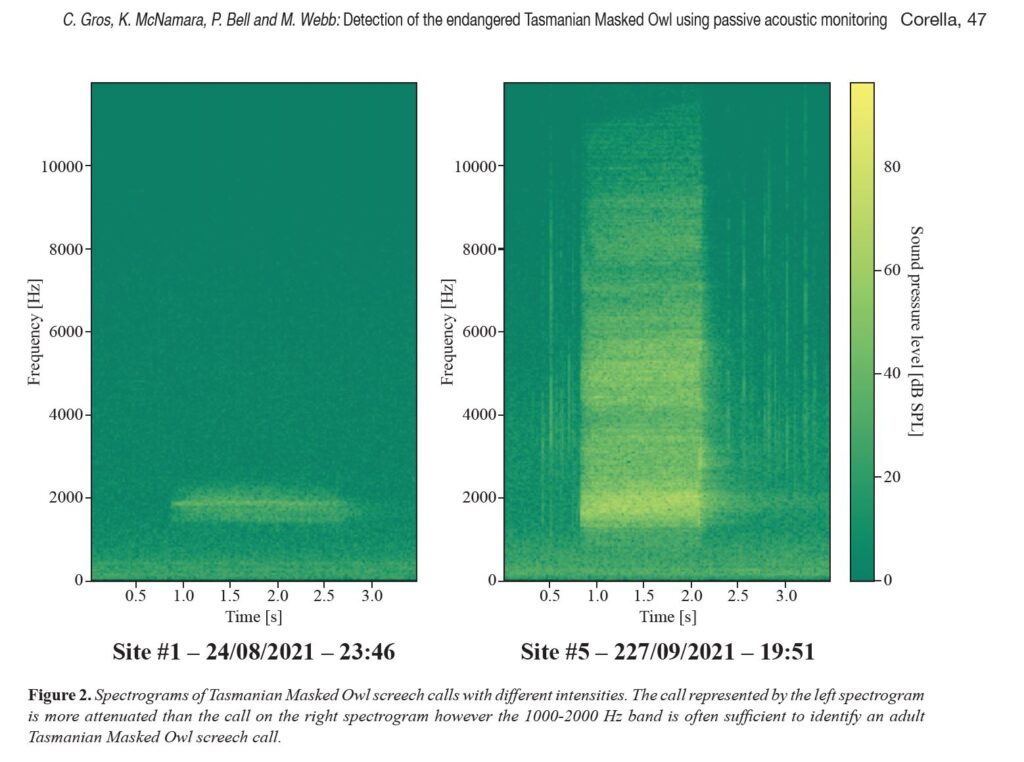
Screeching calls of an adult Tasmanian masked owl can be heard in the audio above
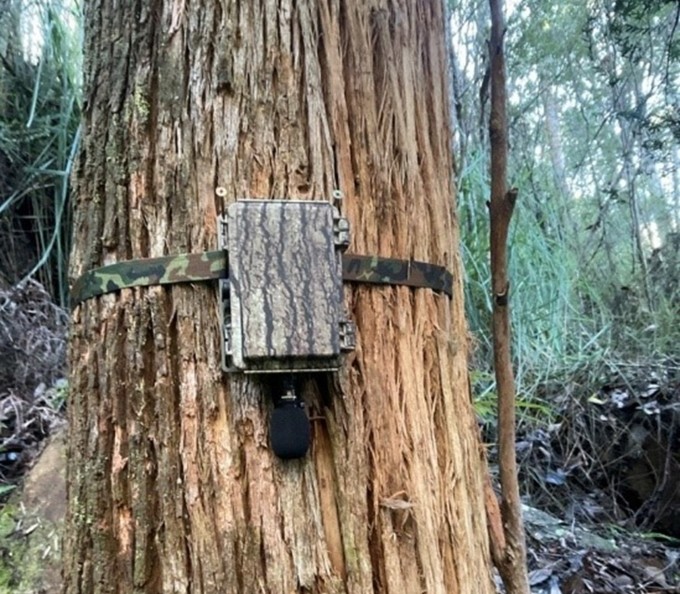
Wildlife Acoustics Song Meter SM4 deployed by Entura ecologists in north-west Tasmania, within a patch of tall eucalypt forest assessed to be potentially suitable nesting habitat for Tasmanian masked owls
eDNA, barcoding and metabarcoding
Increasingly rapid and relatively cheap DNA sequencing techniques are also transforming biodiversity research. Environmental DNA (eDNA) is genetic material from the hair, skin, urine, faeces, gametes or carcasses of organisms that can be found in the environment. This eDNA data can be interpreted through ‘barcoding’, which uses species-specific tools to detect the DNA fragments of a single species within an environmental sample, as well as ‘metabarcoding’, which can simultaneously detect millions of DNA fragments from the widest possible range of species. eDNA barcoding is particularly useful for detecting invasive, rare and cryptic species in places that are otherwise difficult to survey.
What’s next?
Fauna survey methodologies are evolving fast. Soon we’re likely to see continuous, automated wildlife detection and species identification, with solar-powered detection units (camera traps, bioacoustic recorders, etc.) autonomously uploading data to the cloud. This could produce high-resolution activity maps that update in real time and at large scale. Systems that can compute and upload data autonomously and are self-sufficient in energy will allow us to obtain accurate and extensive information from almost anywhere, anytime.
So, are clever bots and gizmos going to take our jobs? Will we never head out into the field with our binoculars again? Not quite yet (happily!), but with increasingly robust hardware, modern computing power and machine-learning, we can do more for our clients and our planet, and that’s a great win–win for us all.
If you’d like to talk with Entura about our ecological monitoring services, contact Raymond Brereton.
About the author
Dr Carley Fuller is an Environmental Consultant at Entura. She is an ecologist with expertise in environmental impact assessments for renewable energy projects including solar, wind, hydropower, hybrid, and transmission infrastructure developments. She has a decade of experience working in multiple Australian jurisdictions and internationally in the United States, Latin America, and the Pacific as both a research scientist and consultant. Carley has a strong technical background in plant science, land-use planning, GIS and natural values assessment and completed her PhD in conservation science at the University of Tasmania. She is passionate about leveraging environmental data to provide tailored decision support for a range of stakeholders.
MORE THOUGHT LEADERSHIP ARTICLES
Understanding the challenges of medium-sized power systems
Power systems in the range of 200–500 MW face unique challenges, including how to incorporate increasing amounts of intermittent inverter-based renewable energy, such as solar PV and wind generation. What are these challenges, and how can they be solved?
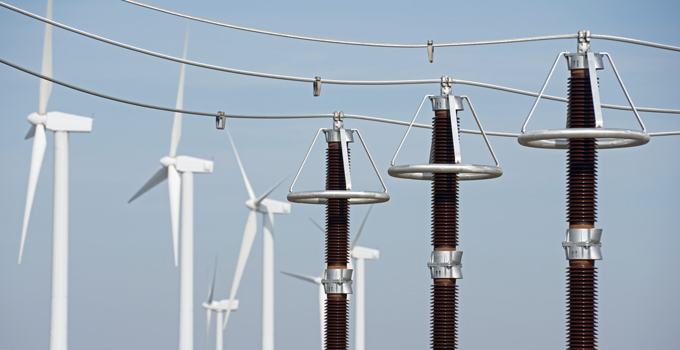
Large power systems, like the interconnected grid of the eastern Australian states, are well-understood. These systems have extensive engineering support and sophisticated models to handle renewable energy integration, with network-wide inverter-based renewables (IBR) penetrations ranging from 25–50% and local penetrations up to approximately 115%. Similarly, small power systems, such as those up to 30 MW found in remote mining sites, also manage high IBR penetrations, sometimes reaching 100%.
However, power systems in the range of 200–500 MW face unique challenges. We call these systems ‘anti-Goldilocks’ power systems. Stemming from the children’s story of ‘Goldilocks and the Three Bears’, Goldilocks has come to mean something neither too big nor too small, neither too complex nor too simple – in other words, ‘just right’. An anti-Goldilocks system, on the contrary, has an uncomfortable combination of both large and small system challenges without the solutions available to a large system operator.
Examples of anti-Goldilocks (AG) power systems in Australia and the Pacific include:
- Fiji power system
- New Caledonia
- French Polynesia (Tahiti)
- Guam
- PNG (Port Moresby)
- Darwin Katherine interconnected power system
- North-west minerals power system (Mt Isa and surrounds)
- Western Australian north-west interconnected system
- the Tasmanian power system during low demand.
Common challenges in AG power systems
AG power systems share characteristics that make managing high IBR penetration both inevitable and challenging.
- 1. Geographical distribution and stability
In small power systems, all generation sources are often close together, ensuring good transient stability. Large systems benefit from high interconnection levels that couple machine inertias effectively. AG power systems, however, are geographically spread out without these stabilising features, leading to difficult transient stability conditions.
- 2. Environmental conditions and storage
Small systems can install enough battery energy storage (BESS) to manage fluctuations in renewable energy sources. Large systems distribute IBR across vast areas, minimising localised impacts from wind and irradiance. AG systems, however, typically have most IBR within a 100 km radius, which means that similar environmental conditions can affect all IBR at once, potentially causing sudden shortfalls in generation.
- 3. Rapid changes in IBR penetration
AG power systems often have high electricity costs and small sizes relative to each IBR station. This makes renewable generation very attractive financially, and a single IBR connection can immediately cause significant penetration increases, potentially reaching 80%+ quickly and catching network operators off guard.
- 4. Responsibility for quality and ancillary services
Because small systems typically have just one generator and one consumer, they tend to have straightforward responsibility allocation for the quality of supply and ancillary services. Large systems are either government-owned or regulated with established market mechanisms for these services. AG systems may lack these structures, often having multiple generating companies and consumers, complicating the provision and funding of necessary services.
- 5. Modelling and planning
Large systems have developed accurate models over many years. Small systems manage with less detailed models because most errors don’t significantly impact overall accuracy. AG systems typically have poor models. The requirement for greater accuracy is only a recent phenomenon, but a greater level of accuracy has been difficult to achieve due to the lack of collaboration between customers and generators, a lack of necessary modelling skills, and a reluctance to see modelling as core business.
Transitioning to inverter-based renewables: four horizons
Successful operation during the transition to IBR involves navigating 4 distinct horizons:
- H1: conventional dominance
The network is dominated by traditional plants with control based on speed and voltage droop. The system can manage almost indefinitely without wide area controls during disturbances.
- H2: high IBR penetration (60%)
There is a high level of IBR penetration, say 60%. While the distributed versus wide area control issues don’t change significantly, prolonged outages of wide area control cannot be tolerated. Systems should operate without human intervention for at least 20 minutes during such failures.
- H3: minimal rotating machines
There are periods with only one large rotating machine. Planners should ensure the system can operate for 20 minutes without human intervention if this generator fails.
- H4: full IBR operation
The system operates with 100% IBR and should be designed to manage without human intervention for 20 minutes during wide area control outages.
Solutions and optimisations
AG power systems face significant but solvable challenges as IBR connections increase. While installing sufficient battery capacity and running rotating plants at low output or adding synchronous condensers can help, these solutions can be costly. Therefore, optimising solutions to minimise additional costs is essential.
Entura has worked on most of the AG power systems listed above and we have found that batteries, while helpful, are only one part of the solution. Effective rules and regulations that allocate risks and responsibilities appropriately, along with a causer-pays mentality and prudent risk acceptance, lead to the more cost-effective technical solutions.
To discuss how Entura can help you ensure the safety of your electrical assets, contact David Wilkey or Patrick Pease.
About the author
David Wilkey is the Senior Principal, Grid & Power, at Entura. David has more than 25 years’ consulting experience across a wide range of electrical engineering projects, including power system studies, power system and generator protection, generator connection rules, and primary plant electrical engineering. David’s primary interests include all aspects of electrical engineering for hydropower projects, such as hydro turbine governors, generator excitation and generator protection systems.
MORE THOUGHT LEADERSHIP ARTICLES
Bifacial solar PV: shining light on all the angles
In the booming global solar industry, installation of bifacial panels has been rapidly overtaking conventional monofacial modules, particularly in utility-scale projects but increasingly at smaller scales (<5 MW) too. But are they the right technical investment for your solar project – and what do you need to consider?
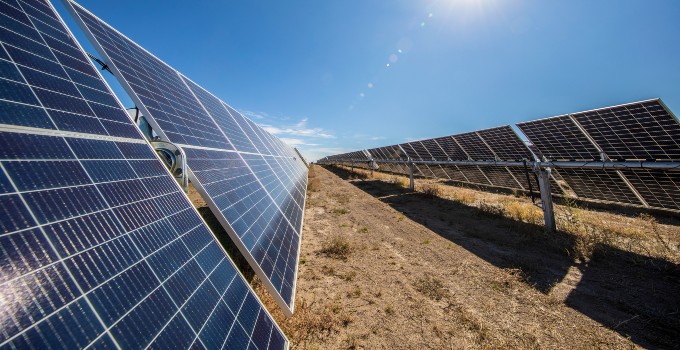
We recommend getting to grips with the benefits, constraints and implications of bifacial modules as early in the development cycle of a project as possible. Here are some observations to get you started.
What are the advantages of bifacial solar PV?
Bifacial solar PV modules are solar panels capable of generating electric current from both sides of the panel, as opposed to monofacial panels, which generate from one side only. Sunlight can pass through a transparent top layer and be absorbed by the solar cells, while sunlight reflected off surfaces can be captured through the transparent bottom layer, increasing the overall power output and potential energy yield.
The advantages of bifacial solar modules include:
- enhanced energy yields (typically 5% and can be up to 10% when optimised at particular sites) with only minor differences in supply cost
- lower levelised cost of energy (LCOE) with greater return on investment (ROI)
- increased duration of maximised power export
- enhanced performance in diffuse light conditions, such as when it is cloudy, which can be beneficial for the stability of hybrid power systems
- greater power density achieved in space-constrained sites
- better end-of-life outcomes, as glass is more readily recyclable than plastic polymers used for the backsheet of monofacial modules
- some manufacturers also claim improved durability and longevity of panels due to double glass construction rather than the glass and polymer backsheet of monofacial modules. This is claimed to be more resistant to environmental factors such as moisture, humidity and fluctuations in temperature. It has also been anecdotally suggested that the glass backface increases protection from water ingress and resistance to corrosion.
Are there any potential downsides?
Bifacial modules typically have a front-side glass thickness of 2 mm with 2 mm on the rear side, compared to monofacial modules which have 4 mm on the front side only. This can increase susceptibility to hail damage, which may require further mitigation measures in hail-prone areas and could increase the cost of insurance.
What’s albedo and why does it matter?
The more reflective a site, the better its prospects for gaining the bifacial edge. Generally, there is a linear correlation between the ground reflectance conditions (albedo) at the site and the power gain from the backside of the bifacial panels. Albedo is also the single largest factor driving bifacial gain.
But a site’s ground conditions will change over time, so one of the most important considerations when calculating the possible benefits of deploying bifacial over monofacial solar modules is determining what the long-term average albedo is at the site. Many factors can play a part in the way the albedo is modelled – including the intended use of the site once the solar plant is built, revegetation strategies, grazing livestock, the frequency of droughts and flooding events, precipitation volume and water pooling, how green the grass is, and the colour of the earth. The highest albedo factors and bifacial gain will be in conditions such as frost or snow, with its high level of reflectance. The lowest albedo factors are achieved on surfaces such as dry asphalt or grasslands.
Is more height a good thing?
Another major factor driving bifacial power is the height of the installation. Bifacial power gain increases with installation height as a greater angle is available for reflection of direct and diffuse irradiation to the rear side of the modules. This gain is most prominent typically between the installation heights of 0.5 and 1 metre before levelling out above 2 metres. In areas prone to flooding, higher installation may also provide extra resilience to increasing weather extremes.
An important consideration here, however, is that although higher installation may increase energy yield and financial returns, there may be considerable additional capital costs and greater complexity of construction of the mounting infrastructure, particularly for longer piles.
What’s the right ground cover ratio?
When the percentage of area covered by PV modules increases, the bifacial gains decrease. If more ground is covered, more area is shaded, and there will be less reflection to the rear side of PV modules. Often there is an incentive for developers to maximise the solar DC power capacity of a given site to avoid costly additional land agreements and minimise the project footprint. However, this can result in a high ground cover ratio (GCR) which can cause shading between rows. This increase in ground shading reduces backside power and energy yield gains (although it can sometimes be mitigated by the ‘backtracking’ capability of single-axis trackers).
Recently, we have been seeing developers take a more conservative approach with this in mind, preferring a GCR below or approaching 0.30.
What about shade from the mounting structure and cables?
Increasingly, manufacturers of mounting structures are looking towards maintaining structural integrity of their equipment while also minimising shading. String cabling can also be a cause of rear shading, so they should be fixed underneath the torque tubes of single-axis trackers (SAT) or underneath the mounting structure supports to minimise any impact. We are noticing an increasing focus on consistency of construction in this regard and the inclusion of this check on installation test certificates as minor shading on one module has the cascading effects of derating the entire string of modules.
Could spikes fry the electricals?
Although asset owners are most interested in the potential for greater energy yield from bifacial modules, it is necessary to also assess the electrical maximum power point voltage and current limits caused by spikes during high irradiance events. These spikes can be caused by a range of environmental factors which may be specific to sites. These include early morning frost at low temperatures, increasing sunlight irradiance at the edge of lensing clouds (magnifying glass effect), snowfall or flooding/water pooling.
In some areas which experience high ground albedo in conjunction with technical designs for favourable backside power gain, the maximum instantaneous bifacial gain can be as much as 15 to 25% for some Australian contexts, which can impact the allowable number of modules in a string as well as the input parameters to combiner boxes, inverters and cables throughout a project.
What’s next under the sun?
Solar is an exciting sector of rapid, continuous innovation, so there will no doubt be ongoing technological evolution with new implications and applications to explore. Regardless of whether bifacial panels are right for your project at this stage, it’s worthwhile considering all the options that might work best for your site. In the transition to net zero, every solar installation has a crucial role to play. The better the yield and value that can be achieved from a solar project, so much the better for the developer, the community, our environment and the future.
If you need support to assess energy yield, design, and technical considerations for your solar project, please contact our business development managers, Patrick Pease (Australia) or Shekhar Prince (international).
About the author
Lachlan McKenna is a renewable energy engineer in Entura’s renewables development team. He works on solar, wind and BESS projects from concept and design through to operations and repowering in locations throughout Australia and the Indo-Pacific region. Prior to working for Entura, Lachlan gained experience in the commercial and industrial rooftop solar sector and European offshore wind industries.
See our previous articles on how to achieve solar success:
Ten tips for developing your engineering career
From Baby Boomers to Gen Alpha, the generation names and characteristics come and go – but despite the changing working styles and preferences of older and younger engineers, some things stay the same. Developing good engineers still calls for many elements that have shaped countless careers over time: people who were willing to share their knowledge and experience, opportunities to develop and refine the engineering craft, and mentors to support us, believe in us and help us make the next step.
I’ve been reflecting on these dynamics at this senior point in my 34-year career – and I’d like to share some tips to help set younger engineers on a path towards achieving a satisfying, successful career.
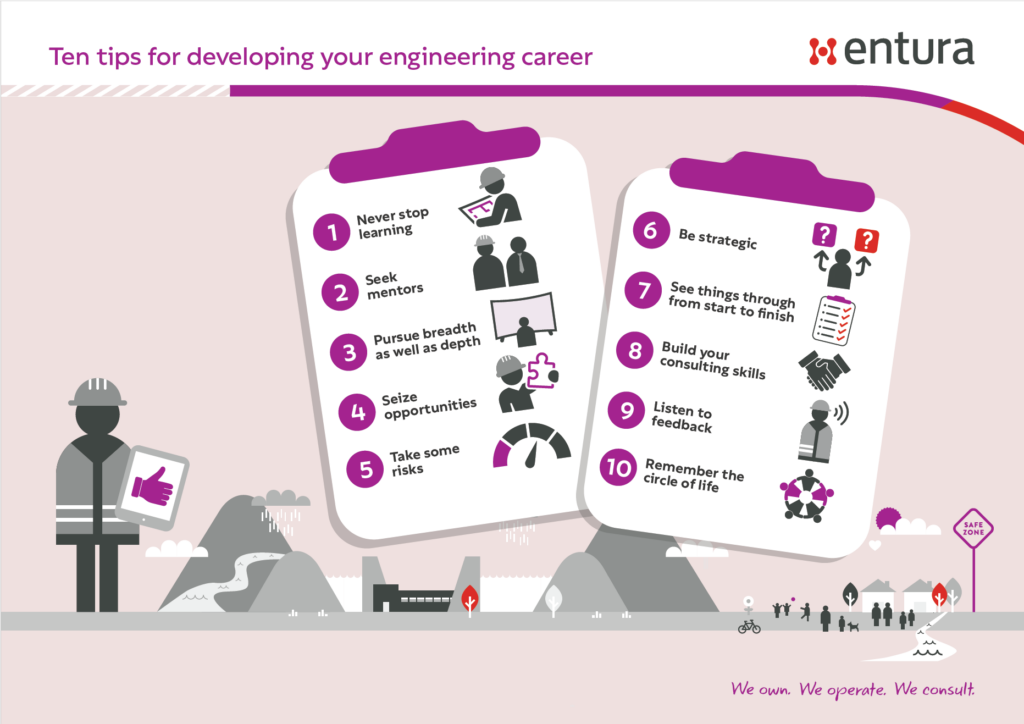
Tip #1 – Never stop learning
Graduating with a formal engineering qualification is only the first milestone in your learning. Explore whether there are postgraduate courses that can help you grow and open up opportunities that interest you. It isn’t easy to balance postgrad studies with work – let alone with the family responsibilities that many people experience in their early/mid adulthood. You’ll need to think carefully about how much time you can devote – and how to maintain a healthy work/study/life balance.
Also look at what your workplace can offer in terms of internal programs, such as broad-based leadership programs. An industry body will often offer short courses and will also provide networking opportunities where you can learn from other people – so join a professional association. Beyond formal courses, you can use your development plan to your advantage by identifying areas that interest you and seeking variety in the kinds of tasks and projects you are assigned.
Whatever career stage you’ve reached, stay interested, interact, and keep asking questions. It’s a great antidote to becoming a ‘know it all’ or getting stuck in a rut! At the end of each year, ask yourself, ‘What have I learnt that’s new?’ If you can’t think of anything, then maybe you’re playing it too safe and it’s time to change things up a bit.
Tip #2 – Seek mentors
Mentors – whether formal or informal – can give you technical insights and can also help guide your broader professional journey. Use mentors to extend your learning beyond your allocated tasks, such as how to be a good consultant, or just broaden your understanding. Think broadly about who you could seek out as mentors along your career journey. For example, some of the members of independent review panels have become de facto mentors to me. Value your mentors, and try to give something back or pay it forward to the next young engineer.
Tip #3 – Pursue breadth as well as depth
Breadth is as important as depth. Try to achieve more breadth before you specialise, because breadth will make you a better expert (where you have depth) and extend your value as a consultant. For me, experience in designing and constructing dams and hydropower as well as stints in hydrology and modelling gave me a more holistic understanding of dam projects. Try to get some experience in other related disciplines, so you are better placed to manage multi-disciplined projects; and get some construction experience so you can see how your designs translate on the ground.
The value of broad experience is evident in the 16 competencies set out by Engineers Australia for ‘Chartered Engineer’ status. Use them to work towards becoming chartered – a target that every engineer should strive for.
Tip #4 – Seize opportunities
Only you can act to take the opportunities that emerge in your career, to make the most of them, and to learn from them. If you think too long, the opportunity may disappear or someone else may seize it. This will sometimes require sacrifices – such as periods away from home, which can be hard – but sometimes a little adversity can really spur your professional and personal development. Opportunities could be a particular project, an opportunity to work with someone you want to learn from, or an interesting career episode in a different place or a different role.
Tip #5 – Take some risks
If someone you respect believes you can do a role on a project, maybe you should too. Stretching yourself will help you develop. Jumping – or being thrown– into the deep end can be a great way to learn, as long as you’re supported so you don’t sink. Talk to your mentors and managers about how they can support you to thrive rather than flail. Remember that mistakes and failures are not the end – they are excellent stimulus for learning, and you certainly won’t be the first to experience them.
Tip #6 – Be strategic
Your employer’s responsibility is to create an environment in which you are able to develop, but ultimately your career is your responsibility. What do you need to learn or achieve in order to get where you want to end up? How can you position yourself so that you’re ready when opportunities emerge? For me, this was the need to have a Masters degree to take on team leader roles on bank-funded international projects – which spurred me to return to study. You could use the competencies for Engineering Chartered status as a benchmark to identify gaps and then work to fill them.
Tip #7 – See things through from start to finish
Look for opportunities to be involved in a project from inception through to commissioning. You will learn a great deal from seeing how the investigations and decisions taken in the design play out in the actual conditions on site as well as the constructability and the performance of the structure. These experiences will shape your expertise, how you operate in the future as an engineer, and the advice you give your future clients. This is equally relevant for other programs of work, seeing the program from a conceptual stage to an operational stage.
Tip #8 – Build your consulting skills
If you want to work in consulting, you need to become more than a technical expert. An ideal consultant needs technical expertise, but also needs to be able to engage effectively with clients, to communicate well (both in writing and orally), to be creative and solve problems, and to manage and deliver projects. These skills are valuable for everyone, regardless of your role. Taking up different roles through your career can also help you see things from different perspectives and become a better consultant. Every experience helps to build the consultant you become.
Tip #9 – Listen to feedback
Even if it’s uncomfortable to receive, seek out feedback and use it constructively to learn more about yourself, your skills and how you interact with others. Everyone has facets in their knowledge, performance and personality that can be enhanced. The more you can see yourself through the eyes of your colleagues, the better you’ll be able to play to your strengths and work on your weaknesses. In the end, many engineering projects require a team to deliver, so if you know your strengths and weaknesses, you can create a balanced team that capitalises on the synergies.
Tip #10 – Remember the circle of life
What goes around comes around. In the early stages of your career, it’s natural to expect support and development. Eventually, as you progress, your expectation should shift to helping develop others. I believe that this cycle should be faster than most people would expect. You don’t need to wait decades. Once you have been doing something for a few years, you can help others, and by doing so you will reinforce your learnings and improve your ability to explain complex technical elements. Developing others will also develop you.
I hope that other Baby Boomers and Generation Xs are inspired by these tips to reflect on your own experiences, share your recipes for success, and look out for where you can help others grow. It’s in all of our interests for the engineering profession to thrive.
Head to our careers page for current opportunities at Entura.
About the author
Richard Herweynen is Entura’s Technical Director, Water. He has more than three decades of experience in dam and hydropower engineering, and has worked throughout the Indo-Pacific region on both dam and hydropower projects, covering all aspects including investigations, feasibility studies, detailed design, construction liaison, operation and maintenance and risk assessment for both new and existing projects. Richard has been part of a number of recent expert review panels for major water projects. He participated in the ANCOLD working group for concrete gravity dams and was the Chairman of the ICOLD technical committee on engineering activities in the planning process for water resources projects. Richard has won many engineering excellence and innovation awards (including Engineers Australia’s Professional Engineer of the Year 2012 – Tasmanian Division), and has published more than 30 technical papers on dam engineering.
MORE THOUGHT LEADERSHIP ARTICLES
Powering a greener mining future with hybrid renewables
This article reflects on a panel discussion chaired by Ray Massie at the Energy and Mines Australia Summit 2023, which you can read more about here.
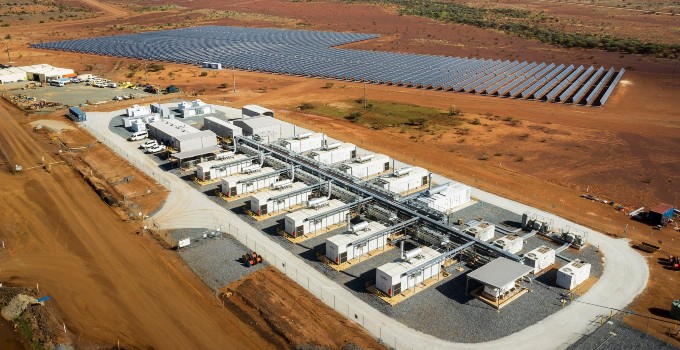
The discussion about powering mines with renewable energy has moved a long way in a short time. As the imperative to go green has escalated, the mining sector has grown hungrier for viable, rapid and cost-effective strategies to decarbonise, build greater social licence and tap into the competitive market for products with a lower carbon footprint. Integrating cheap, abundant renewable energy into mining operations is the natural solution.
These are exciting times as the sector moves far beyond the ‘why’, ‘whether’ and ‘when’ of renewables – and digs much, much deeper into the nuances of ‘how’.
Early forays into small hybrid off-grid renewable energy systems have demonstrated and built confidence in the technology – so there is no longer any question in the mining industry of whether off-grid hybrid renewable systems will work. Off-grid hybrid projects aren’t small test beds anymore. They’re ground-breaking, large-scale, cutting-edge renewable power systems of the future, able to be deployed on a fully commercial basis with clearly understood risks and operational adjustments. In many ways, the mining sector is out ahead of the pack, and the deployment of renewables on off-grid mine sites is offering lessons for the wider power sector and the future of the grid.
With many of the early technical risks resolved, the driving focus now is how to take full advantage of what a hybrid system can offer. How can the system be optimised to maximise its benefits? What’s the required level of reliability? How much storage is enough – and in what form?
Of course, every mine site is different, so there are no one-size-fits-all answers. And today’s answer may not be the right fit for tomorrow, given the rapid and continuous transformation of the energy landscape – in terms of technology advances, policy shifts, price volatility and global trends. It’s a dynamic space.
Integrated control and storage change the game
Traditionally, the relationship between mining operations and power supply has been a relatively simple transaction requiring a given amount of power with a set level of reliability and availability. The modelling of the power system was based on simple load metrics and power quality dictated by the capabilities of thermal generation plant. This equated to a very simple, flat cost of energy with any variance at the macro scale driven by changes in the price of fuel.
As we all know, when we switch over to multiple intermittent and variable generators, things become more complex. One of the keys to unlocking the benefits of a hybrid renewable system is the integrated control approach. With the correct control philosophy in place, you have many more ‘levers’ that can bring each element into play for a given operational mode or system event. This enables achievement of the levels of power quality and reliability that are needed, rather than being limited to the levels that traditional thermal plant could supply.
With this sophistication of control, we can start to think differently about reliability across the whole mining operation. Does the mining plant process or works need the same reliability or availability across everything all the time? Perhaps, for example, power supply for pumping may not require as high a level of reliability as other more critical areas of the plant. A clear understanding of the true reliability needs will help derive the optimal design at minimal cost.
When storage comes into the mix, we can also start to think differently about how to best match energy supply, timing of energy-intensive activity, and storage of excess energy to get maximum value from the renewable resource and minimise both the cost of energy across the operation overall and the cost per tonne of the mine’s output. The economics of long-duration storage are improving all the time.
New horizons for energy storage
Although the technology and the sector have travelled a long way already, there are still issues to finesse. One area of uncertainty is the optimal and most cost-effective storage technology and duration.
Going back a decade, the economic storage duration was 10 to 20 minutes, using the battery primarily for its discharge capability. Now we are seeing economic storage durations approaching 4 hours, which lend themselves to energy-shifting roles in which the charging capability comes into play as well as the discharge mode. The economics of longer duration storage will continue to improve but the optimum arrangement will vary site by site.
Funding for advancing novel long-duration energy storage (LDES) technologies has increased by 36 times over the past 5 years[1]. While lithium-based batteries still dominate and are likely to continue to do so for the foreseeable future, many more types of storage are now possible, including metal-air, pumped hydro, compressed air, flow batteries, gravity, thermal and various novel chemistries. Some storage technologies require specific topography and geology, for example pumped hydro and large compressed air storage, and others are at varying stages of technical readiness. Flow batteries of various chemistries are experiencing a significant amount of support aimed at breaking through the long-term storage cost barrier, though this is yet to be achieved at scale, and more likely to be competitive at longer durations (>10 hr) and in larger sized systems (>100 MW).
It is clear, however, that finding the best LDES for a mine project is not just for the mining sector to solve alone. The entire global power industry is looking at storage – and the mining sector can take full advantage of this as LDES evolves. What the mining sector can do right now is to identify the energy use and demand side opportunities that various storage durations could unlock for their projects.
Another key learning we have found over years of hybrid system development is that not every bit of energy produced by renewables needs to be stored. Some can be spilt or, better still, adjustments can be made on the load side to better match the variability in generation. Once the system stability, reliability and demand-side opportunities have been addressed, the storage of ‘spill’ really comes down to economics, which will change over time as storage costs decrease.
Collaboration and risk management
With all these new approaches and possibilities – and the many factors that always come into play such as mine life, capital limits and risk – it’s possible to over-analyse. Not every new project needs to break barriers and set new records. There is definitely still a place for choosing the low-hanging fruit, as the primary case for renewable hybrids being cheaper than thermal-only is well established. You will never really be able to answer every question in a single project – or even predict every question that might arise.
This is where you simply don’t have to go it alone. The key to overcoming hesitation is collaboration. Getting the correct advice is always worth the investment, as is sharing learning at an industry-wide level. There are many players that specialise in one or more aspects of the future mix of technology, mining process, hybrid power operation, renewables knowhow, integration skills, commercial thinking and so on – and bringing these skills together is a must.. Collaboration is the key to solving problems, reducing risk and its appropriate allocation, and a successful project that will benefit all industry players more than competition and working in isolation.
We also believe in learning by doing, through delivery of a specific project. Forming a group of key parties to drive forward a tangible project makes it far more manageable. In innovative projects, ‘pushing risk through’ often just comes back as significantly increased cost. A better approach in these cases is a shared risk profile. The learning is a significant part of the return in early adoption.
Are the right people and the right materials available?
The industry is already experiencing a shortage of experienced hybrid system operators. This needs to be managed through increased training and backup. We believe that operational personnel should be engaged very early in the design of a hybrid power system so that they can have input into development of the system, gain familiarity with it, and consequently feel a level of ownership of the assets.
It is also important to have a close relationship between those involved in the mining process and those operating the power system. For the power system operators, this is a chance to better understand the criticality of various mine plant processes; for the mine operators, it’s a chance to get a better understanding of the mix of operation modes that can be used to meet the mine’s changing power needs.
In many ways, the people skills and resources needed across all the project delivery stages (design, deployment, operation, maintenance and support) are more important than the technology – and this needs to be considered carefully for hybrid projects to be successful.
Finally, neither the mining industry nor the energy sector at large will be able to transition to renewables if we don’t have the right minerals and materials on hand to manufacture clean energy technologies such as wind turbines, solar panels and batteries. Having the mining industry supply these resources in a sustainable way is a powerful contribution to the clean energy transition.
ABOUT THE AUTHOR
Ray Massie has more than 30 years of expertise in renewable energy technology. He managed the development phase of the Hydro Tasmania iconic King Island Renewable Energy Integration Project (KIREIP) and Flinders Island Hybrid Energy Hub as well as undertaking key roles for hybrid system projects at Coober Pedy, Rottnest Island and more recently EDL’s Agnew Hybrid Renewable Microgrid powering Gold Fields’ Agnew Gold Mine and Scott Base in Antarctica.
Entura is an expert in hybrid off-grid renewable energy systems, from our world-leading King Island Renewable Energy Integration Project through to large-scale off-grid mining solutions such as EDL’s Agnew Hybrid Renewable Microgrid, powering Gold Fields’ Agnew Gold Mine. If you would like to talk to us about integrating more renewables and storage into your energy equation, contact Patrick Pease, Donald Vaughan or Greg Koppens.
[1] “The long and the short of energy storage tech”, Climate Tech VC (CTVC), March 2023
MORE THOUGHT LEADERSHIP ARTICLES
Monitoring tailing dams with unified data visualisation
This article is based on a recent presentation by Richard Lindqvist, a data integration specialist with Entura, at the ANCOLD Tailings Dam Operators Forum 2023. Richard describes the need, opportunities and barriers to adoption of advanced monitoring systems.
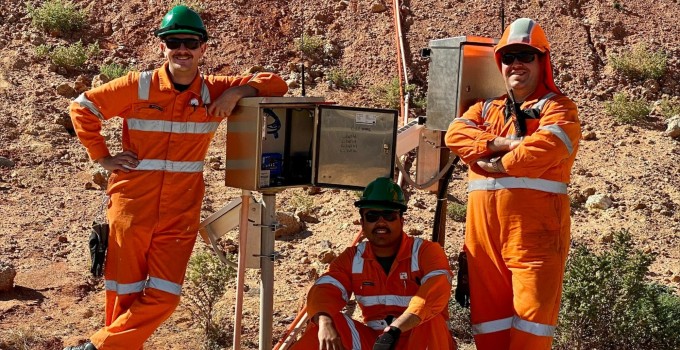
Tailings facilities are crucial for preventing any spread of mine tailings into surrounding land and waterways. Some tailings dams are among the largest engineered structures in the world. Like all dams, they must be managed to protect downstream communities and the environment from harm.
The catastrophic collapse of a tailings dam in 2019 in Brazil focused intense global attention on the safety of tailings facilities. In 2020, a new Global Industry Standard on Tailings Management (GISTM) was released.
The global standard emphasises the need for appropriate monitoring of tailings facilities throughout their lifecycle – from design to closure – to aid in the mitigation of risk.
Advanced monitoring systems can assist greatly in meeting and exceeding the requirements of the GISTM. Here we’ll discuss the potential of telemetry, automation and meaningful visualisation of monitoring data – and the benefits that can flow from implementing such a system at your site.
The GISTM sets the context for monitoring
Accurate, meaningful and continual data from monitoring systems forms an important part of a comprehensive and integrated knowledge base, which is a core principle of the GISTM, particularly with respect to Principle 7 of the standard.
Monitoring data is to be assessed at the frequency recommended by the Engineer of Record (EoR). Any conditions exceeding the dam’s critical operating parameter ranges (COPs) must be addressed promptly through trigger action response plans (TARPs) or critical controls.
The COPs are parameters that if exceeded have a real risk of leading to a dam safety incident. Each COP has a TARP. Careful and regular monitoring of COPs can identify early signs of a potential safety issue and allow rapid intervention before the trigger level is reached.
These requirements set the context for monitoring methods, and reinforce the importance of accurate, up-to-date, meaningful information that tells a clear and timely story about the facility’s current state, trends and potential concerns.
New technologies have changed the face of monitoring
As technology has progressed, new opportunities have arisen for monitoring tailings facilities, with enormous benefits in efficiency, immediacy and accuracy. Now, COPs, TARPs and data quality can be assessed in near-real-time using automated processes – enabling efficient and early identification of faults, such as during construction activities. Time spent collecting field data and maintaining systems is minimised, and the saved time can be reallocated to dam inspections and deeper assessment of data trends. End-to-end connectivity allows multiple sources of telemetry data and operational data to feed immediately into a unified and user-friendly visualisation platform, supporting understanding and decision-making. Monitoring data can also feed proactive and predictive maintenance in asset management and operations.
Opportunity abounds, but barriers remain
As with any new standards and systems, there are likely to be some barriers or challenges in moving from a design or concept to reality. In our experience, some common factors include resistance to change, the potentially significant cost to invest in the new technology needed for an efficient and integrated system, and the balance of resourcing between automated versus field data collection and processing. Other common hurdles are integration with existing systems and workflows and the data security and governance standards to be met. Staff training and succession planning can also be a challenge but are essential to maintaining the necessary knowledge base.
None of these challenges are insurmountable – and the effort to overcome them will be repaid by the immense benefits in operational intelligence, efficiency and reliability that advanced, automated, integrated systems can deliver.
A real-life example of an evolving monitoring system
Entura has been on a journey with a large mining client for nearly 15 years, continually improving the facility’s monitoring solutions. Initially, we used our own Ajenti telemetry loggers and Ajenti Data Management System (ADMS) before progressing to the integration of other vendors’ logger data to the ADMS. We are now moving forward in using OSI Pi to integrate a broad array of data sources for our clients, presenting them within an accessible and adaptable visualisation platform.
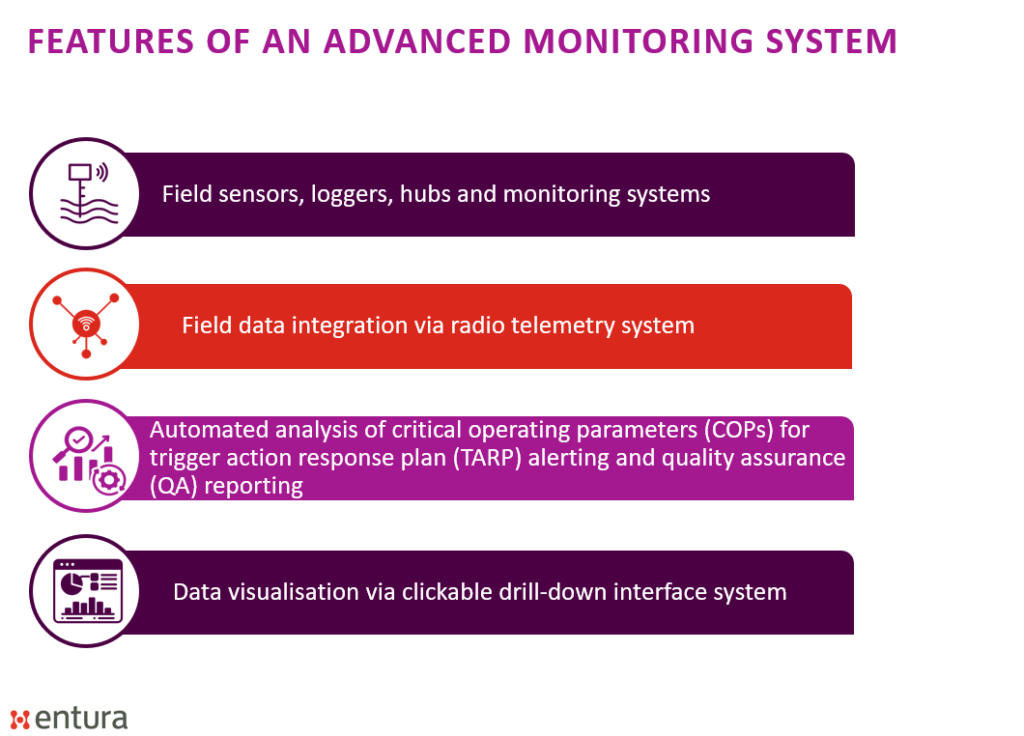
Historically, data was retrieved manually from field loggers for processing into spreadsheets and manual analysis of charts. With data retrieval via telemetry, along with automated analysis and visualisation, this time can now be spent reviewing TARP alerts and in-depth assessment of data.
System reliability and robustness has been another key factor in our work with our clients – with improvements such as fit-for-purpose enclosures (316 stainless steel), solar power installation and the ability to assess operational data such as battery voltage and signal strength. This is reducing the physical maintenance needs at monitoring sites and replacement of enclosures or batteries. The focus can now be on routine inspections of dam conditions rather than managing the monitoring network.
Effective record-keeping is essential when managing a large monitoring network. The new system enables a rich knowledge base to be compiled, stored and interrogated. Site metadata and documentation can be hosted on the visualisation platform, including calibration and installation reports, FATs and SATs (factory and site acceptance tests), analysis reports, memos, photos, field observations and general site maintenance requirements. Data from various field note sources (i.e. ArcGIS, fieldmaps, Iauditor and Fulcrum) can be brought into the visualisation system for ease of access and asset management. Electronic field note apps are used on mobile devices so that data can be recorded in the field and synced directly to the system.
We have also added value to the data repository by incorporating other technologies into the integrated system and visualisation interface. These include aerial survey overlays, weather monitoring, soil moisture sensors, camera imagery and operational data – and we are always working with our client to ensure that visualisations are intuitive and easy to interpret.
The visualisation interface provides real-time and trend data for users, and can hone in on individual sensors anywhere across the facility. Faults can be analysed onsite using analysis tools – and offsite using available data trends.
We’ve also worked with our client to provide a secure data ingress pathway for remote equipment, and we’re continuing work on integrating the new system with existing traditional and emerging SCADA systems.
Throughout the long evolution of this monitoring journey, we have continually added new innovations to best serve our client’s needs – and are always looking for new ways to deliver effectively.
Every system needs continuous improvement
The existing system has brought significant benefits to our client’s ability to analyse and visualise the facility’s data. Yet technologies continue to improve and evolve at speed. For our clients, we are now using OSI Pi and Pi-Vision as a primary integration and visualisation tool, and have developed a proof-of-concept for delivering the capabilities of the current system using OSI Pi and Pi-Vision. This will allow greater flexibility to bring on-site data into a single visualisation system – agnostic of the historical databases currently in use.
With the pace of technological advances likely to accelerate, clients and consultants should all stay attuned to new monitoring developments and alert to possibilities for improving their system’s functionality and integration with a broad range of sensors and control systems.
With the right data at the right time coupled with meaningful analysis and user-friendly visualisation, every tailings facility’s management should be better able to make earlier and more informed decisions to reduce risk.
To find out more about Entura’s data and water management solutions, contact Phillip Ellerton.
MORE THOUGHT LEADERSHIP ARTICLES
India and Australia can support each other’s clean energy journeys
The relationship between India and Australia goes far beyond our nations’ mutual love of cricket. We have deep people-to-people connections that have been formed over generations. At the beginning of the 20th century, around 7000 people of Indian descent lived in Australia. Now, Indian-born Australians number more than 750,000 – a number that has doubled over the last decade and continues to grow.
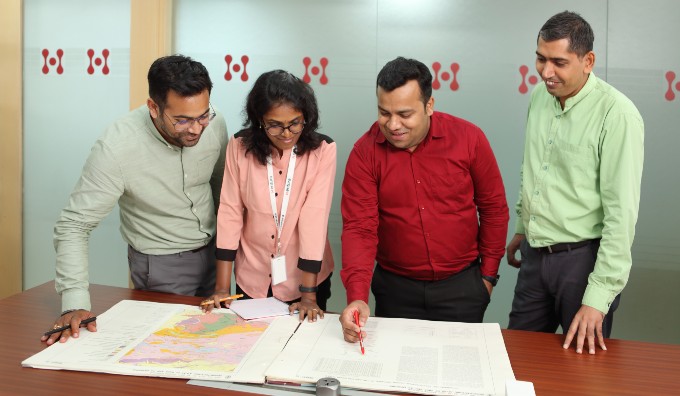
It’s natural that our two countries should have a strong relationship across many domains. We have strong strategic and political ties, and our economic engagement opportunities are massive. This will be strengthened even further with the Australia-India Economic Cooperation and Trade Agreement.
In a sign of the deepening India–Australia relationship, in 2023 Australia’s Prime Minister Anthony Albanese and India’s Prime Minister Narendra Modi have shared senior bilateral visits both in India and in Australia. One of the major themes in these meetings has been the potential for the two nations to support each other’s journeys towards a clean energy transition and net zero targets.
Building clean energy connections
I’ve been privileged to be involved in furthering the Australia–India relationship as a member of the Australian Senior Business Delegation to India and a subsequent Roundtable with business leaders in Sydney. During these meetings, I spoke about the potential our nations have, despite our radically different sizes, to work together to overcome challenges in our renewable energy sectors. I put forward the idea of a joint renewable energy council, which was well received. I am now on the Australia India CEO Forum’s Energy, Resources, Net Zero and Critical Minerals Joint Working Group, which had its first meeting this month. And this week, Entura has welcomed a visit from the High Commissioner of India to Australia, Manpreet Vohra, at our Hobart offices.
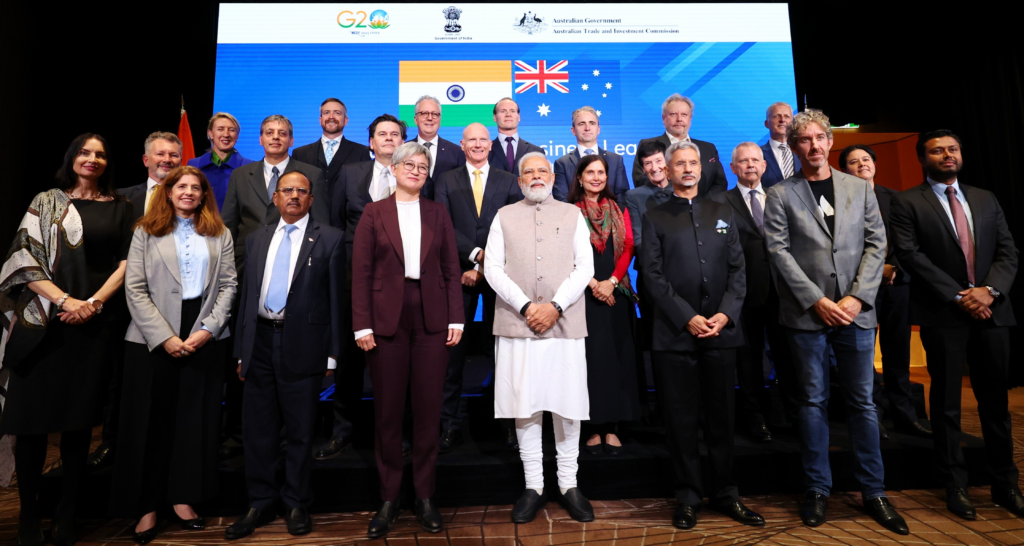
These opportunities have been realised after forging relationships with government officials across Australia and India over many years – and having maintained an Entura presence in India for more than 17 years with our highly skilled team based in New Delhi. Entura is a leader in the renewable energy industry and at present is the only Australia-based, government-owned business operating in the sector in India.
Entura is an excellent example of how Australia and India can support each other towards our clean energy goals. With India committed to net zero by 2070 and with 60% of its current energy production coming from coal, there are huge decarbonisation moves underway, including plans for 500 GW of renewable energy by 2030. Renewable electricity is growing at a faster rate in India than in any other major economy. Electricity demand is expected to double over the next decade and the share of wind, solar, hydropower, batteries and pumped hydro will lift as a proportion of the mix.
For Entura’s clients in India, we bring deep hydropower and pumped hydro capability and significant expertise in hybrid renewables systems, integrating distributed energy resources, resolving transmission challenges, and delivering bespoke, tailored training and capacity building through the Entura clean energy and water institute, with a particular focus on dam safety. For our projects in Australia, our India team brings a wealth of talent and a boost to capacity. They also have the local knowledge and connections that go a long way towards our success in clean energy and water projects across South Asia and South-east Asia.
Building on Entura’s long engagement with India, and from my involvement with the International Hydropower Association, I see the following areas as particularly important for our nations to focus on for mutual benefit in the energy transition.
1 Diversifying supply chains in critical minerals and renewable energy technologies
As both countries strive to achieve ambitious renewable energy targets, we will need to develop more diverse and resilient supply chains. The extraction and processing of critical minerals will be an essential area for cooperation. Mechanisms such as The India–Australia Critical Minerals Research Partnership and India–Australia Critical Minerals Investment Partnership will integrate Indian scientific, industry and government partners with CSIRO’s developing Critical Energy Metals Mission. This holds great potential to strengthen supply chains, add value to Australian exports, and support the commercialisation of critical minerals technology.
Another crucial factor in the success of Australia’s renewables, green hydrogen and green steel ambitions is the availability of components such as solar PV panels, batteries, electrolysers and electrical componentry. India is a powerhouse of manufacturing capability and could become a major supplier of these essentials to support Australia’s build-out of renewables. The announced joint taskforce on solar and the initiatives underway to collaborate on green hydrogen and green steel are encouraging developments.
2 People power and knowledge-sharing
Developing a skilled workforce, ready to take up the jobs of the future, is a make-or-break factor for the clean energy transition. As Entura has experienced, India has a deep pool of technical talent in renewable energy and a rising skilled workforce. To put it in context, India has over 1000 universities and some estimates suggest that these institutions are producing more than 1 million engineering graduates per year.
Australian businesses such as Entura benefit from having access to this talent – especially facing escalating skills and resources shortages in Australia – and we’ve found that with the right tools, methods and attitudes, our people across Australia and India can work seamlessly together in integrated teams, leveraging each other’s strengths on projects throughout the Indo-Pacific. Our India team adds diversity and capacity to our business – with the added bonus of optimising project delivery across time zones. We also benefit from the ability to build greater understanding of local conditions and regulations in India, and to forge strong networks and partnerships in the region.
Over the years, we’ve identified that despite the size of the potential renewable energy workforce, India still seeks greater skills development and expertise in hydropower, dam safety and the integration of renewable energy technologies. Entura’s clean energy and water institute (ECEWI) is a model of how long-term experience of developing and maintaining renewable energy assets, which we’ve gained through Tasmania’s century-long hydropower journey, can be shared across the world to build skills and capacity that extend far beyond the theoretical or academic.
Through ECEWI, we’ve delivered successful exchange programs, training workshops, and capacity-building initiatives in India, including dam safety training for India’s Central Water Commission as part of its Dam Rehabilitation and Improvement Project (DRIP) – and we’re now supporting the South Asia Regional Infrastructure Connectivity Framework (an initiative of the Australian Department of Foreign Affairs and Trade) by providing capability development on dam safety and cross-border power markets.
3 Innovation and best practice
There is enormous scope for Australia and India to work together to drive innovation in clean energy technologies and to share best practices and solutions. Many initiatives are already underway, drawing on Australia’s advanced technical expertise in solar and wind farms, energy storage solutions, and grid integration projects. For example, Entura is applying experience in India that we’ve gained in world-leading projects in Australia – such as our understanding of offgrid hybrid renewables systems gained from powering the Bass Strait islands, and the work our teams have led to identify and progress the first new pumped hydropower opportunities in Australia for decades in Tasmania and in Queensland.
As developers conduct concept studies and seek to secure licences for renewable projects, sharing international expertise such as ours will be an advantage. We are already applying our pumped hydro screening processes to identify new opportunities in India, and looking at the potential for repurposing disused mine sites for new renewable energy projects, as we are doing at the former Kidston gold mine in Queensland. We’re also applying our knowledge of repowering existing wind and solar farms in the quest for economical and sustainable approaches to boost electricity generation.
This brings me to another key area in which Australia can help to support a ‘fair’ clean energy transition in India. Australia has some of the strongest environmental and social requirements for clean energy projects in the world, and we’ve learned a lot about sustainability along the way. Entura has long been an advocate for sustainable projects conducted with integrity. In fact, I’ve just returned from the International Hydropower Association’s world congress, which reinforced this theme, announcing a new Hydropower Sustainability Alliance and the Bali Statement of Powering Sustainable Growth. Businesses like Entura can foster a fairer clean energy transition in India by promoting high standards of sustainability in all the projects in which we participate and the training we deliver.
Working together on the three factors I’ve discussed here will help build greater success, resilience and sustainability in all areas of the renewable energy transition. Lowering emissions in the global energy sector is an enormous and daunting endeavour that can’t be solved without international collaboration. But we’re at a very exciting point in the journey. We look forward to continuing the close and mutually beneficial relationship between our nations. Accelerating the global clean energy transition is the most important legacy we can leave today’s communities, future generations, and this planet we all share.
About the author
Tammy Chu is the Managing Director of Entura, one of the world’s most experienced specialist power and water consulting firms. She is responsible for Entura’s business strategy, performance and services to clients, and is part of Hydro Tasmania’s Leadership Group. As a civil engineer, Tammy specialised in the design and construction of mini-hydro and hydropower systems, project management, hydropower investigations, prefeasibility and feasibility studies, environmental assessments and approvals, resource investigations and resource water management. Tammy is a member of the Board of the International Hydropower Association. She was the first female and now past president of the Tasmanian Division of Engineers Australia, and was an Engineers Australia National Congress representative.
MORE THOUGHT LEADERSHIP ARTICLES
Who will develop and build our clean energy future – and what will it cost?
I have just ‘returned’ from the World Wind Energy Conference 2023, held just down the road from Entura’s Hobart office. At the conference there was an overwhelming sense that the energy transition from fossil fuels to renewables is well underway and the era of coal-fired power generation is coming to a close.
This has been reflected in the Australian Energy Market Operator’s most recent roadmap (the 2022 Integrated System Plan) which shows the need for a phenomenal increase in energy storage (batteries and pumped hydro), wind and solar generation. AEMO estimates that, by 2050, storage will need to increase by a factor of 30 (from 2 GW now to 61 GW in 2050), and that grid-scale solar and wind will need to increase 9 fold (from 16 GW now to 141 GW in 2050). Take a look at AEMO’s infographic which makes the scale of the energy transition challenge very clear.
This generational change offers many opportunities and challenges for the industry and the wider community. But technical challenges aside, the huge scale of what is needed has me pondering two non-technical questions: who exactly is going to develop and build so many projects, and what will it cost?
Who is going to do the work?
Regarding my first question, I think of the varied background of my colleagues and imagine where the next generation of engineers might come from. At Entura, we have renewable energy experts including an electrician turned civil engineer turned renewable energy engineer who is now pursuing his passion for battery projects and electric vehicles. We have a former manufacturing engineer, now with 15 years of experience as a wind engineer. We have an expert on space tether dynamics, now one of Australia’s most respected solar and battery experts. And we have an electrical engineer from the oil and gas industry who is now helping design and commission hybrid energy systems from outback Australia to Antarctica. The common thread is that we work with people who were willing to change track and pursue what they believe to be the new right course of action.
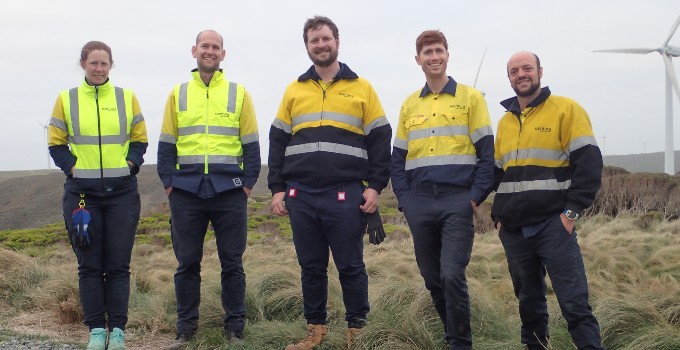
For renewables to achieve the necessary growth, we are going to need people from diverse technical and cultural backgrounds. Those of us in leadership positions need to be willing to employ new graduates and support people who may initially lack specific experience but will in short time become valuable contributors.
At the conference, Kane Thornton (Chief Executive of the Clean Energy Council) called on leaders in the industry to put aside their own propensity to try to carry the load, and instead focus on developing new leaders as a priority. With such ambitious targets for new renewable energy, this is the right, future-oriented approach. We all have a part to play, and the theme of the conference, ‘Symphony of the Renewables’, points to a collaborative, industry-wide approach rather than a zero-sum project-vs-project approach.
What will it cost?
On the question of cost, CSIRO (in collaboration with AEMO) issues an annual estimate of the cost of different forms of energy generation (GenCost: annual electricity cost estimates for Australia – CSIRO). This study suggests (with evidence) that wind and solar backed by storage technologies will be the cheapest way to replace coal-fired plants and meet new demand as our country grows.
This is worth celebrating, but the industry must also prepare society for the large expenditures needed to rebuild our energy system, and the cost increases that consumers may sometimes see in their energy bills. While wind and solar are comparatively less expensive than new coal, gas or nuclear, it will not be cheap to achieve the necessary build-out of wind, solar and energy storage that will takes us towards 100% renewable energy. By design, this system will have some infrastructure that will be idle during times of plentiful wind or sun but is vital to the security and resilience of the system overall. This will be a challenging feature of the system to communicate to people who might see in any given year an increase in their household electricity bill.
The overall message needs to be communicated, repeated and reinforced – that this is a once-in-a-lifetime rebuilding of our electricity system into something that is more reliable than we currently have, ready for the future, and cleaner and better for our towns and communities and the wider world.
If you would like to find out more about how Entura can help you optimise your wind farm, develop an asset management strategy, or support you with due diligence services for proposed or operational projects, contact Patrick Pease or Silke Schwartz on +61 407 886 872.
About the author
Andrew Wright is Entura’s Senior Principal, Renewables and Energy Storage. He has more than 20 years of experience in the renewable energy sector spanning resource assessment, site identification, equipment selection (wind and solar), development of technical documentation and contractual agreements, operational assessments and owner’s/lender’s engineering services. Andrew has worked closely with Entura’s key clients and wind farm operators on operational projects, including analysing wind turbine performance data to identify reasons for wind farm underperformance and for estimates of long-term energy output. He has an in-depth understanding of the energy industry in Australia, while his international consulting experience includes New Zealand, China, India, Bhutan, Sri Lanka, the Philippines and Micronesia.
MORE THOUGHT LEADERSHIP ARTICLES
A fast and fair energy transition demands a skilled, diverse workforce
The clean energy transition is set to create millions of new renewable energy jobs worldwide. It will, however, also bring major change for workers and communities who currently depend on carbon-intensive industries.
This leads to two major challenges:
- how can the clean energy sector find enough skilled workers to enable the mammoth task ahead of us?
- how can we make sure that no one is left behind so that the transition is both fast and fair?
These are enormous questions for the clean energy sector as a whole, not for hydropower alone. All players in the sector need to own our part of the challenge and act urgently to find and implement the solutions that are right for us.
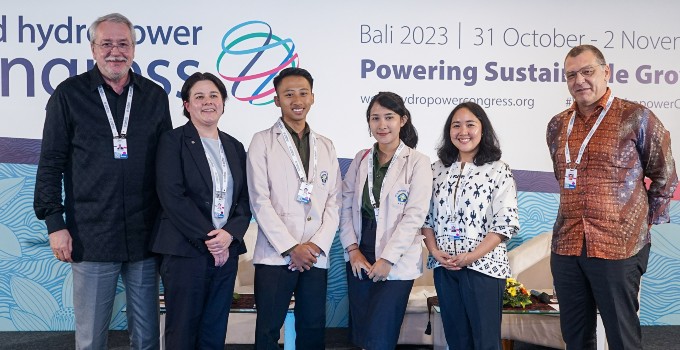
At the recent International Hydropower Association Congress in Bali, I facilitated a panel discussion on these issues. With hydropower globally already experiencing staffing and skills challenges, our panellists agreed that the sector needs to plan and act now on at least five fronts:
1 Retaining organisational knowledge
The sector will need a strong focus on knowledge transfer, especially as talented and deeply experienced professionals retire from the industry. It’s estimated that as much as 25% of the hydropower workforce will reach retirement in the next decade, taking their knowledge and skills with them.
2 Attracting and retaining a skilled workforce
All clean energy technologies will be competing for talent as the energy transition accelerates towards 2050 net zero targets. Hydropower will need to consider how to remain competitive in this tight employment market. The fact that hydropower plants are often located in remote areas can be a barrier to some people. Scholarships, subsidies and payment levels may help to offset some of the barriers, as will creating supportive, flexible and safe workplaces with attractive terms of employment.
There’s also a need to ensure, and promote, hydropower’s environmental and social sustainability, as more and more workers are increasingly motivated by purpose, values, integrity and sustainability. It’s important that we demonstrate how a career in hydropower can be enormously fulfilling, with so many opportunities to make a meaningful difference to society and a sustainable future.
Another important consideration is that we’re not talking about engineers and electricians alone, although priming the pipeline of STEM students and apprentices is particularly urgent in these areas. We’ll also need a vast range of other professionals, with skills in finance, law, project management, people management, IT, environmental science, planning, community engagement and more. Workforce planning needs to take these role into account.
3 Activating a range of options for skills development and training
We will need to ensure that we’ve got the right mix of different training options available – from formal tertiary and vocational training, through to customised and on-the-job training (such as the skills and capacity development that Entura’s clean energy and water institute provides) and less formalised upskilling. The next seven years will be critical here, so that the workforce is ready as we hit the major escalation of clean projects in the race towards 2050.
4 Mapping opportunities in the sector to support a ‘just transition’
What can we do to find mutually beneficial opportunities for workers and communities affected by changes in carbon-intensive industries? The transition to clean energy industries isn’t necessarily a straightforward switch for workers (for example, are the jobs of the right quality, in the right places, and at the right time?) – but could hydropower be an option for some? How can we support displaced workers to adapt to the jobs of the future? To drive policy, countries need to move quickly to analyse their current and future workforce capacity and needs, and their transition challenges and opportunities – as Australia is doing. Take a look at the recent report from Jobs and Skills Australia, The Clean Energy Generation: Workforce needs for a net zero economyand, to see what’s happening in this space around the world, read the International Energy Agency report on Skills Development and Inclusivity for Clean Energy Transitions.
5 Maximising women’s participation in the sector
It’s well recognised that women are still underrepresented in engineering, in the overall clean energy workforce, and in hydropower. The gender gap becomes larger at senior and managerial levels. Women also leave the hydropower sector more often than men (whether that’s changing sectors, changing companies, or leaving the workforce altogether). As I’ve said many times before, our industry simply cannot afford to miss out on the talent of half the population. We need greater action to maximise opportunities for women and other underrepresented groups in the hydropower workforce and access the widest pool of diverse talent.
So what can we do about this? We can:
- address the ‘pipeline problem’ – by raising the profile of STEM as a path for schoolgirls/ university students / apprentices
- create incentives to attract women into the industry (e.g. scholarships)
- ensure unbiased recruitment approaches
- adopt flexible, supportive and equitable workplace strategies to help attract and retain women in the industry, particularly women balancing caring responsibilities
- ensure that hydropower workplaces are secure and safe for women with appropriate facilities
- fix the pay gap
- create opportunities for networking and mentoring to encourage career progression and industry retention
- ensure that women are encouraged to apply for senior positions, and that women in senior roles are visible role models for others
- involve men in understanding and remediating existing gender barriers – a particularly powerful action, as identified in the recent World Bank report on gender equality in the hydropower sector
- think, talk and act on gender issues every day, not only on International Women’s Day!
In short, there’s no lack of action we can take to make the hydropower sector more inclusive and diverse, even if those actions look a little different or take more time in different regions. The most important thing is to move forward.I strongly recommend that you read Power with Full Force: Getting to Gender Equality in the Hydropower Sector, which has just been released by the World Bank. It’s a powerful study of these issues, and, for me particularly, a strong reminder that the conditions we might take for granted in countries like Australia, such as paid parental leave and anti-discrimination laws, are not enjoyed by all women in the global hydropower sector, particularly in developing countries.
Clearly, to secure the next-generation workforce needed to deliver the renaissance in hydropower, we’ll need to work together to tackle the challenges and make the most of the opportunities ahead. None of the five actions I’ve listed in this article are surprising and none are merely a ‘nice to have’ – they are all essential and urgent.
Talking about these issues at global conferences is important, but it’s not enough. Now is the time for meaningful and urgent action to turn observations into actions and intentions into results.
We would like to acknowledge the panel participants: Kate Lazarus, ESG Advisor – IFC, Martin Stottele, Team Leader of RESD (Renewable Energy Skills Development, Indonesia) Programme, and Josef Ullmer, Director Andritz Hydro, Andritz.
Click here for more information about the Entura clean energy and water institute. For more information on our work in sustainability and planning, please get in touch.
About the author
Tammy Chu is the Managing Director of Entura, one of the world’s most experienced specialist power and water consulting firms. She is responsible for Entura’s business strategy, performance and services to clients, and is part of Hydro Tasmania’s Leadership Group. As a civil engineer, Tammy specialised in the design and construction of mini-hydro and hydropower systems, project management, hydropower investigations, prefeasibility and feasibility studies, environmental assessments and approvals, resource investigations and resource water management. Tammy is a member of the Board of the International Hydropower Association. She was the first female and now past president of the Tasmanian Division of Engineers Australia, and was an Engineers Australia National Congress representative.
MORE THOUGHT LEADERSHIP ARTICLES
What is the best way to model complex water structures?
Understanding the behaviour of water as it flows in natural and constructed environments is fundamental to designing and managing many civil assets, and integral to reducing risk and cost in water infrastructure projects.
Water is such an intrinsic part of our lives that we all have a good observational understanding of what water is and what it does in many everyday situations, like falling from a tap or spiralling down a plug hole. But our intuition can only take us so far. When engineers design important infrastructure such as dam spillways, or inlets to pump stations, or need to understand issues such as fish passage for a low weir, they need to turn water into numbers and quantify its behaviour. Being able to quantify complex water flow is one way engineers can better understand the world and make a difference.
Modelling options
When water flow is complex and a deeper understanding of its behaviour is required, the two main options to help improve this understanding are physical scale models and computational fluid dynamic (CFD) models. But when should one be used over the other?
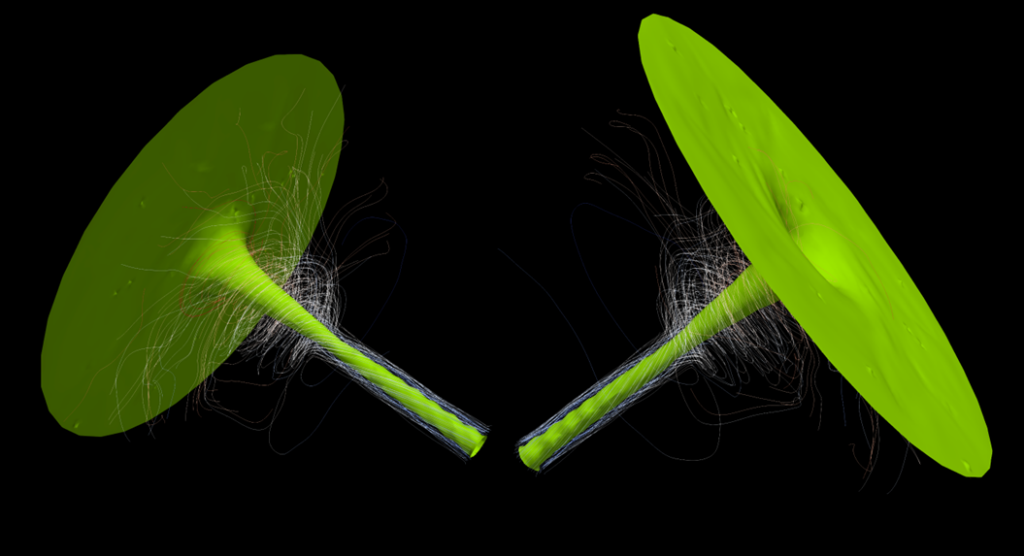
Physical scale models are created and used in a research laboratory, whereas CFD models are created using software and run on fast computers. Here ‘scale’ means the physical models are built at some fraction of the item of interest’s size. Historically, physical scale models have been the gold standard for helping understand complex water flow, but that’s not always the case now. Both methods have a place, and they can complement each other.
It’s generally better to use more advanced modelling techniques early in a project to better guide the design process or understand an existing system.
Has CFD killed the physical scale model star?
Knowledge about the physics of water flow has developed from centuries of physical observation with experiments run in hydraulics laboratories and the field, combined with theoretical calculations. Advances in computing performance in the last few decades have allowed these theoretical calculations to be solved in finer detail and with greater accuracy. But does this mean the end of physical scale modelling testing, in particular as computing power is growing faster than the number of research laboratories? Not for a while.
Even with advanced, powerful computing systems, the outputs of CFD software tools still need to be validated against physical measurements. For the physical scale of the civil engineering problems being solved (e.g. a dam spillway), the CFD models approximate the theoretical calculations, and these approximations need to be validated. Some water flow problems are dominated by well-defined forces, such as gravity, and these problems are easier to validate. But other problems are dominated by turbulence and multiphase flow, and these are harder to validate as the phenomena are more complex.
A note on turbulence and multiphase flow
In the aerospace and car industries, where the fluid of interest is air rather than water, a designer uses a combination of wind tunnel testing and CFD modelling to better understand the performance of a design. Small parts can be simulated in CFD with minimal approximations using direct numerical simulation (DNS) of turbulence. But for a full car and even more so for a large commercial aeroplane, it’s currently cost-prohibitive to only use DNS (e.g. millions of dollars per run), and some level of wind tunnel testing is still required. This is combined with non-DNS modelling CFD methods.
It’s similar in civil engineering. There are different physical sizes, stages of a project timeline, costs, and risks of getting the answer wrong. These drive the balance between CFD and physical scale model testing. Most work, in particular at a concept level, is done with CFD, but physical scale modelling is still recommended for critical infrastructure prior to construction.
A matter of scale
The size of most civil engineering infrastructure – such as spillways, rivers and large stormwater culverts – make it impractical to use DNS of turbulence (based on cost and timing).
Both physical scale models and CFD can be time-consuming tools to use, and for various projects it’s better to use one before the other. For the design of fish passages and recreational water systems (such as whitewater kayaking), doing small-scale, rough physical scale modelling can be efficient for design development of concepts. This is because designers can move 3D-printed shapes around in a hydraulic flume, and see and feel the direct impact on changes to the design. This is a very complex problem with few industry guidelines and without the same body of research as other civil engineering problems, such as dam spillways and energy dissipation.
Where there is a large body of knowledge on a topic, standard designs provide a starting point. Then, it’s often efficient to start with hand calculations or a concept-level CFD model. These calculations may lead to more detailed design, modifications of standard designs with detailed CFD modelling, and then, potentially, physical scale model testing. There are always iterations, and sometimes the CFD modelling can be used to inform the design of a physical scale model testing setup, and vice versa. CFD modelling is also faster and can more easily be used for design optimisation compared to physical modelling.
In some cases, using CFD alone is possible, particularly where there is a solid body of knowledge about a piece of infrastructure. The temptation is to use CFD more and more, as it can often be lower in cost. It is also important to note that physical scale model testing has its limitations. So there are cases where CFD modelling can provide more insight at a lower cost.
With physical scale model testing, the key limitation is in its name. The model is built at a much smaller scale than the feature being modelled – just a fraction of the expected final design or existing system size. Typically a coarse model of a dam or spillway may have a 1 in 200 scale, and a good one may be at a 1 in 50 scale. A 1 in 50 scale model of a spillway that was going to be 100 m high when finally constructed would need to be 2 m high as a model. This is okay for pure water models, but for aerated flow the ideal scale is closer to 1 in 10. So if aerated flow was important in that 100 m high spillway, then the model would be 10 m high. At that size it would not fit in a normal hydraulics laboratory and would be very expensive to build. So, typically, a compromise is made and a 1 in 30 model is constructed. The reason scale is important is that water behaves differently at different scales. For example, surface tension is important in a pipe that’s 5 mm in diameter but is not important in a large river flow.
How do you measure water?
Another limitation of physical scale model testing is measuring the flow during the experiment. There are some limitations on CFD as well but, compared to a physical scale model test, it is generally much easier to get the results from a CFD model accurately, in many more locations, and without disturbing the flow.
Complex models demand high-performance computing
Complex multiphase flows for fish passage and smaller whitewater kayak systems can be modelled well with scale models initially, as the system being modelled is relatively small. But when you’re modelling something that is much larger and has complex multiphase flows, scale model testing is harder. Model size is important for CFD models as well, and there is no free kick here. Larger systems where there is complex flow are computationally more expensive to model in detail using CFD. Typically, however, the budget of projects involving larger complex systems are also larger – and so, the modelling costs can be a similar proportion of the total project costs for small and large projects.
At some point in the future, such as when quantum computing becomes mainstream, CFD using direct turbulent simulation (i.e. DNS) of larger objects could completely replace physical scale modelling.
Room for both in pushing the knowledge envelope
Both physical scale modelling and CFD modelling require specialised facilities and experienced modellers. With the advent of lower cost computing services and increased CFD training in many engineering courses, CFD modelling has become the lower cost option for many civil engineering water modelling problems. But both physical scale modelling and CFD will continue to remain important for many years yet, and both approaches should be encouraged.
In general, what’s easy or difficult in physical scale modelling is often of a relatively similar level of difficulty for CFD modelling. Often the approach will come down to a modeller’s preference and access to either option, but in some cases it’s prescribed by standards. CFD is often cheaper and faster to set up for most civil engineering scale problems and, once set up, can be run on multiple computers at once and address more questions at once. Viewing the results of the CFD model is more flexible, but some prefer the touch and look of a physical scale model.
For novel phenomena at the edge of our understanding, there is still no substitute for a physical scale model. Over time, this will leave physical scale modelling mainly as a research tool and to aid community consultation and education, with CFD used for operational modelling in industry. Most projects will still use a combination of the two.
Like all good engineering projects, solving complex water modelling problems requires having adequate time and budget to provide a suitable quality result. When approaching a complex water flow problem, inform yourself of the solution options. The best model for your stage of understanding is the one that reduces uncertainty and improves knowledge to aid better decision-making.
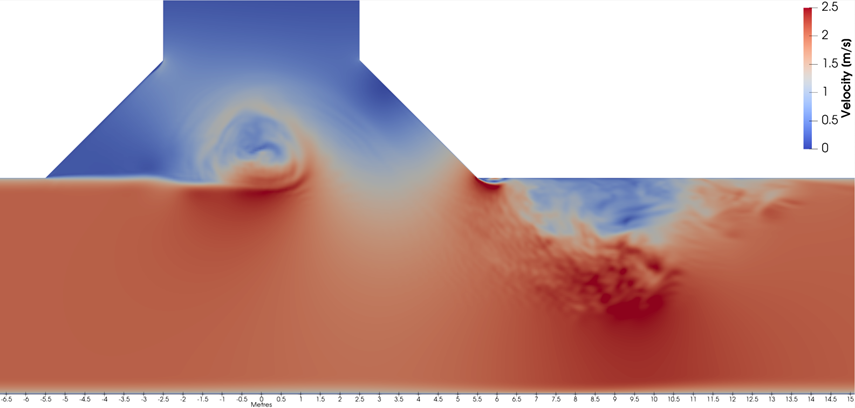
If you’d like to talk with Entura about your water or dam project, contact Phillip Ellerton (Australia) or Shekhar Prince (international).
About the author
Dr Colin Terry is a civil engineer at Entura with three decades of experience in Australia and New Zealand. His experience includes modelling, planning, design and construction support. He has worked on multidisciplinary projects across various parts of the water cycle including catchment management, water supply, hydropower, land development, transport, and water quality in natural systems – with a focus on surface and piped water.
MORE THOUGHT LEADERSHIP ARTICLES
De-risking renewable energy projects is in everyone’s interests
The rapid transformation of the energy sector is exciting and ripe with opportunities. Inevitably, it also involves a level of complexity and a range of uncertainties. Phrases such as ‘decarbonising the future’, ‘net zero emissions’ and ‘energy security’ feature in almost every energy transition seminar, but what do they really mean when it comes to constructing and operating the projects necessary to support a cleaner and greener future? How can developers design and develop for sustainability across the whole project lifecycle?
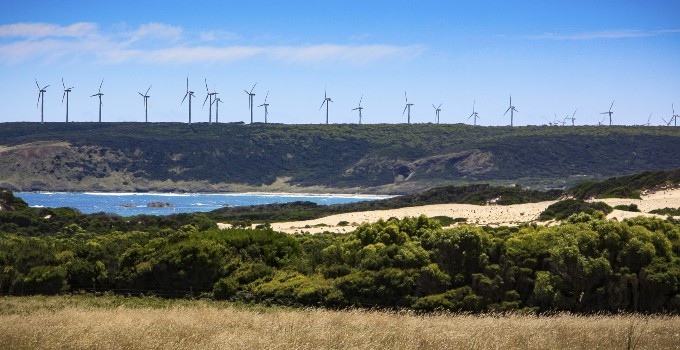
With over 6 GW of committed large-scale wind and solar projects in the development pipeline, many of which need external finance, it is crucial that projects are developed in a way that will qualify for financing and will align with contemporary sustainability standards. Many financial institutions are applying risk management frameworks such as the Equator Principles to determine, assess and manage environmental and social risks. Over 100 financial institutions globally, including key Australian banks such as Commonwealth Bank, ANZ and Westpac, have adopted the principles. The Equator Principles provide a framework for due diligence into whether the project has been or is being developed in an environmentally and socially robust manner including respecting human rights. Similarly, regulators require evidence of sound avoidance and minimisation strategies to reduce the footprint of disturbance, particularly through construction.
Environmental, social and governance (ESG) considerations throughout the whole project lifecycle, including supply chain considerations, are more carefully scrutinised than ever before. Poor management of ESG can lead to reputational, financial and legal consequences. Investors will want a thorough review of approval documentation and evidence of systems and processes to assess project risk regardless of whether projects are already approved or are still under assessment. The investor needs reassurance that the project will be, or has been, developed in a manner that aligns with their own ESG objectives.
To help your project progress smoothly through the approvals process, consider these points:
Each project’s risks, and their relationships, are unique
No two projects are the same. There are myriad ESG risks for any project, and the work to understand and quantify these risks is also project-specific. The interrelationship of the risks influences the risk profile of each project, which is pivotal to investment and acquisition decisions. Some examples of risks include changes in regulation between the time of construction and approval; changes in the conservation status of threatened species that alter operational monitoring requirements; community resistance post-approval; or lack of transparency throughout the supply chain amidst the risks of modern slavery.
Start early with thorough studies and engagement
From an approvals perspective, there is significant benefit in investing early and proportionately in environmental and social studies. It’s also important to invest in well-documented planning assessments that include building a relationship with the regulator as well as robust community and stakeholder processes. Meaningful and genuine engagement with First Nations communities is crucial, as projects are often developed on Country not previously subject to development. In terms of environmental and social risks, some projects may simply be too difficult or inappropriate to develop at the largest possible scale. As the number of projects being developed in a region increases, cumulative impacts are increasingly at the centre of communities’ and assessing agencies’ concerns, particularly in terms of noise, visual impacts and ecology.
Getting on the ‘front foot’ delivers results
It is important for developers and proponents to understand that the quality of work at this early stage is likely to impact on the due diligence process – for example, whether studies have been prepared to best practice or industry standards, and whether risks have been identified early and managed appropriately. Good early studies, actions and decisions can make a difference even before the project is approved – providing regulators with confidence that you’re being proactive when it comes to ESG risks, whether that be through design iterations or measures to manage construction and operation.
Investing early to de-risk may reduce the potential for disproportionate costs later in the project, such as re-work or offsets, and may increase the project’s likelihood of achieving finance. Due diligence throughout the approval process is likely to reassure a potential investor or buyer that the project has been de-risked as early and as thoroughly as possible. With the energy transition such an urgent priority in Australia and across the globe, it’s vital that generation, storage and transmission projects progress both rapidly and sustainably. A focus on early de-risking is undoubtably a key enabler of this goal.
Entura has led a number of supply/vendor and lender technical due diligence assessments nationally and internationally, assisting in the investment, acquisition and transaction for large portfolios of renewable energy projects, including solar, wind and battery energy storage systems. Reach out to Patrick Pease (Australia) or Shekhar Prince (international) to find out more about how we can support you with due diligence assessments.
About the authors
Cynthia Nixon
Cynthia’s experience and qualifications in environmental engineering, environmental law and communications provide an integrated perspective on sustainability risks for Entura’s clients. She has over 15 years experience implementing systems and managing compliance and risk, including auditing, risk assessment, training and reporting. She currently manages Entura’s Integrity Framework and supports clients to improve their sustainability performance. She has conducted due diligence assessments across hydropower, solar and wind farm projects and assessed performance against the Equator Principles and International Finance Corporate Performance Standards (IFC PS). She is a certified user of the Hydropower Sustainability Standard and Tools.
Bunfu Yu
Bunfu is a Senior Environmental Planner at Entura with diverse experience in strategic and statutory planning, environmental approvals and management, and engagement and consultation streams for small to large-scale projects across Australia and the Indo-Pacific. Working with public and private clients across the water, energy and infrastructure industries, she designs and leads fit-for-purpose approval strategies that consider the community, landscape and regulatory regime, and advises on acquisition, joint venture and/or purchase of portfolios in due diligence roles nationally and internationally. She was awarded the 2023 National Young Planner of the Year by the Planning Institute of Australia.
MORE THOUGHT LEADERSHIP ARTICLES
What is the value of conventional and pumped hydro storage in a transitioning energy market?
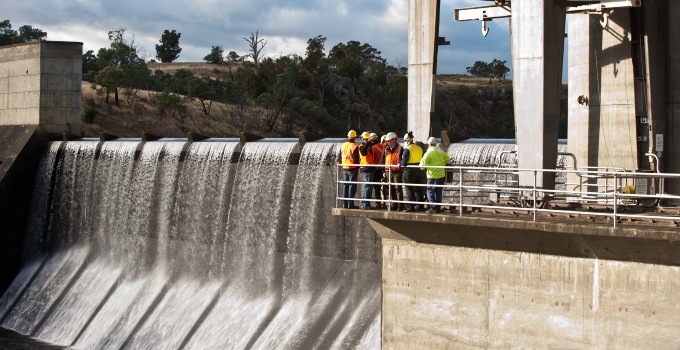
Entura’s Technical Director Power, Donald Vaughan, presented a paper at the global CIGRE Symposium in Cairns, Queensland in September 2023. This is a short summary of the outcomes of the analysis presented in his paper, regarding the value and roles of conventional hydropower, pumped hydro and battery energy storage as the power system transitions to a greater proportion of variable renewable energy in the form of wind and solar PV.
There is a growing need for firming and/or storage in our power systems as we move from predominantly dispatchable sources of power to variable renewable energy (VRE). In the Australian context in the National Electricity Market (NEM), the volume of storage required is expected to be around 46 GW (640 GWh) by 2050 (AEMO 2022). Multiple sources, technologies and methods are being considered but it may be that conventional hydro as well as the more obvious pumped hydro and battery energy storage (PHES and BESS) can play a role.
How do we value storage?
Storage can be valued in many ways. For pure storage, like BESS and PHES, value in a market sense can be measured based on the arbitrage between charging and discharging. Similarly, we can place value on a firming function for VRE. It is more difficult to value the restraint of generation at one time so that stored or withheld energy can be used later. This is the kind of storage offered by conventional hydro sources.
One way of thinking about value is through a measure of realised value vs average value in the market for a particular generator or group of generators. This ‘value factor’ approach is an appropriate measure for storage if we are measuring storage as a contributor to meeting market demand (either local or via interconnections).
One of the problems with the value factor approach is that it is difficult to apply properly without considering market price changes. Introducing plant that can effectively change demand profiles (such as PHES or BESS) will also change the market price, making it difficult to calculate value factors without complex market modelling.
We can instead measure the value of storage by assessing its ability to firm VRE. The measure is based on the ratio of smoothed to unsmoothed VRE with and without the storages applied.
Where there are large amounts of VRE, flexibility from market participants can be valuable. In terms of hydropower generation, conventional hydro plant (storage, Run of the River and head waters) has sufficient flexibility to provide significant market value for modest levels of VRE.
The scale of the transition to VRE will, however, exceed the capacity of conventional hydro to manage variability. Other forms of firming and actual energy shifting, rather than just energy withdrawal (as conventional hydro provides), will be required once the energy balance tips towards energy coming predominantly from VRE sources, with the associated need to manage large diurnal variability as well as longer, weather-based variability. Pumped hydro and battery energy storage will play an important role in providing this more flexible storage.
The role of PHES and BESS in firming
The BESS high power-to-storage ratio allows it to respond to a series of short-lived surpluses or deficits. The PHES deep storage allows it to manage longer duration surpluses or deficits.
The ultimate mix between PHES and BESS in the market is likely to be driven by several factors including the rate of VRE development relative to the level of inter-connection, load growth and VRE mix.
The nature of the VRE will also play a role in determining the storage mix. A predominance of solar will lead to a diurnal pattern that will allow all storage forms to recharge more regularly than would be the case with wind alone. This may allow shallower storage to manage evening peaks with deeper storage reserved for baseload operation overnight.
The economics of BESS and PHES will also play a role in determining the right balance. The market demand for batteries for other purposes and the high market demand for power electronics may yet lead the price/MW of these two storage forms to begin to converge and to limit the depth of storage that will be possible using BESS. PHES on the other hand requires long-term planning, complex approvals processes and less certainty in terms of capturing value from the market. The Integrated System Plan (ISP) of the Australian Energy Market Operator (AEMO) forecasts a large requirement for PHES as deep storage and some very large projects are beginning to be planned.
As these factors play out, the appropriate mix of BESS and PHES will become clearer – but both will certainly play a role in Australia’s clean energy transition.
For more information about the analysis behind his paper, or to discuss how conventional hydropower, pumped hydro and battery storage can be valuable to your situation, contact Donald Vaughan.